Gravitational waves are ripples in spacetime.
According to Einstein’s theory of general relativity, when a mass moves it causes spacetime to curve, like a heavy weight pulling down on a sheet.
But if a mass is accelerating then the curvature is constantly changing, and this change causes waves to radiate at the speed of light.
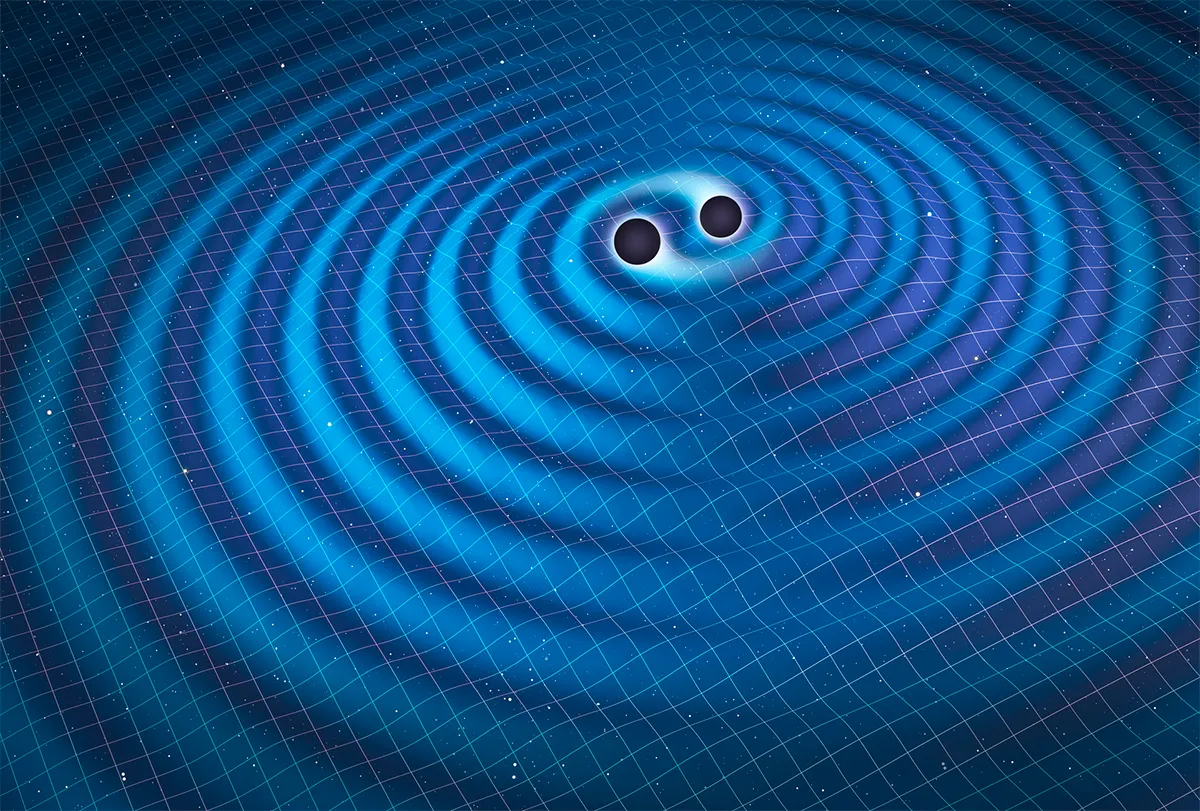
It is these waves that researchers spent decades looking for.
On 11 February 2016, David Reitze, executive director of the US Laser Interferometry Gravitational-Wave Observatory (LIGO), announced that gravitational waves had finally been found.
‘‘We. Have. Detected. Gravitational waves. We did it!”.
This, the first detection of gravitational waves, confirmed a general relativity prediction made by Albert Einstein.
It provided astronomers with detailed information about two colliding black holes and paved the way for a totally new kind of astronomy.
Gravitational waves simply explained

You can visualise gravitational waves by thinking of empty space as a jelly.
If you tap the jelly, ripples are sent through it, alternately compressing and stretching the dessert a little bit.
As a result, the distance between two grains of sugar suspended within the jelly will periodically decrease and increase, despite the absence of any motion.
Likewise, the distance between two points in space will vary slightly as a result of a passing gravitational wave.
Usually, however, the effect is extremely weak: the variations are on the order of a billionth of a billionth of a per cent.
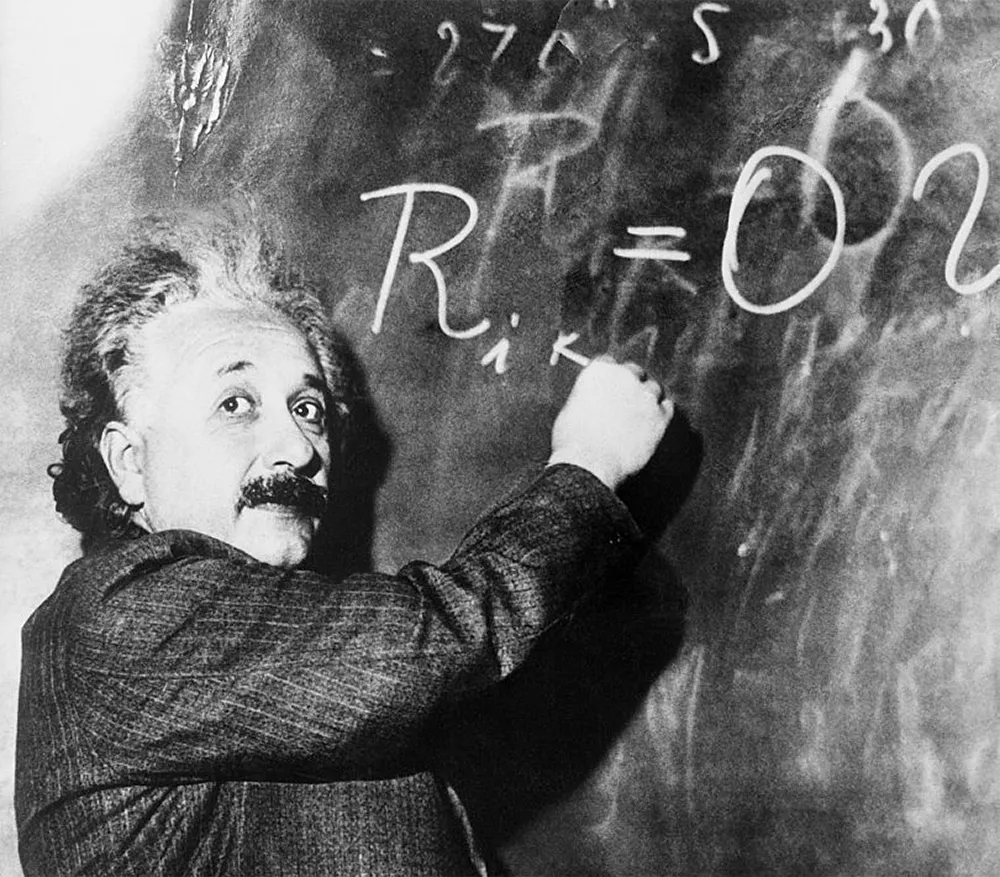
The existence of gravitational waves was first predicted by Albert Einstein in January 1916, just over a month after he published his theory of general relativity.
He theorised that they would be generated by accelerating masses.
Two decades later, in the mid-1930s, physicists still debated whether ripples in spacetime were a physical reality.
In the 1970s, observations of a binary neutron star indirectly confirmed their existence.
The binary lost orbital energy in precise accord with Einstein’s predictions.
It would take another 40 years before gravitational waves were actually detected.

Searching for gravitational waves
Gravitational waves are notoriously difficult to find.
Gravity is the weakest force, and is only influential on large astronomical scales when massive objects are involved.
Looking for tiny changes against this huge background is extremely difficult.
The BICEP 2 mission, for example, peered back to a time a billion trillion trillionths of a second after the Big Bang.
At this point the Universe was much smaller, so gravitational waves had a much larger effect on their surroundings, leaving an imprint that can still be observed 13 billion years later.
Now things are different. Matter is much more spread out, so the effects of these waves aren’t as large.
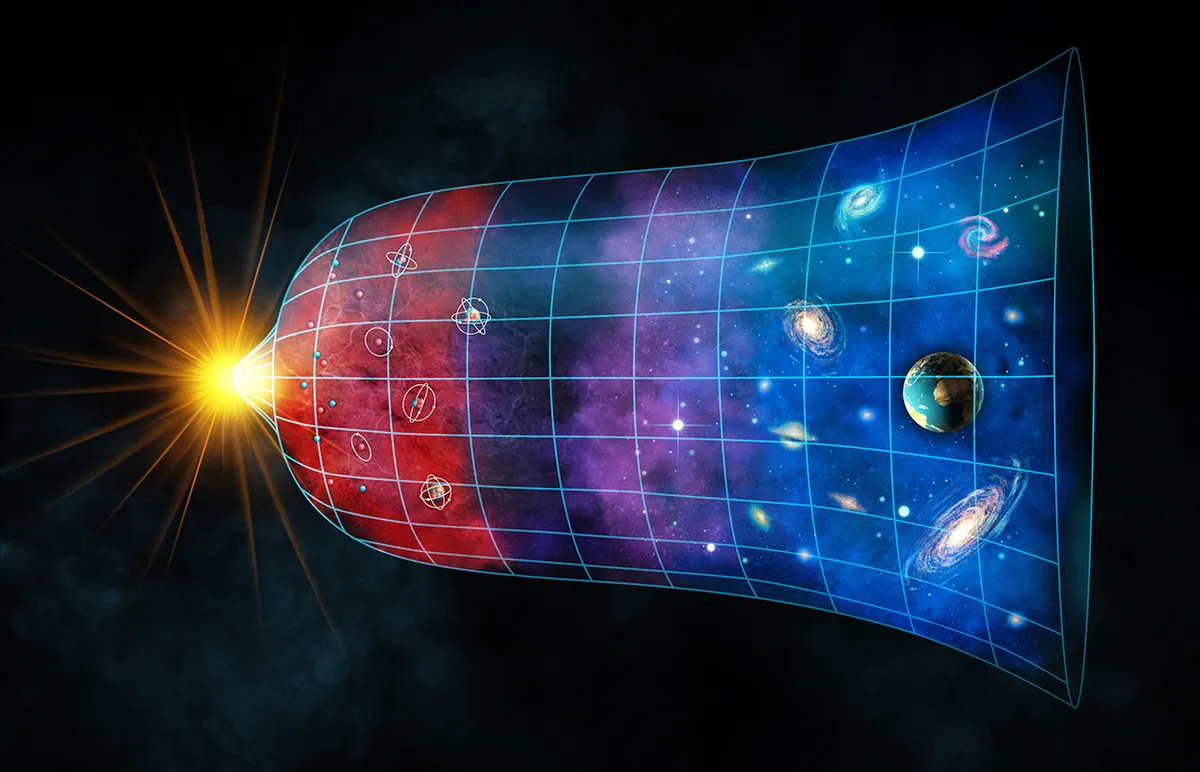
In order to be observed, gravitational waves have to be huge, and these are only created in rare cataclysmic events.
Today researchers wait for an all-important ‘direct detection’; an observation of a gravitational wave from a recent event at the moment it passes through the Earth.
To pick up these waves, scientists built huge detectors called interferometers, which work by using lasers to precisely measure a distance of several kilometres.
As a gravitational wave passes through the light beams, it causes spacetime to shrink and expand, changing this distance by a tiny amount.
It’s this alteration that the researchers are looking for.
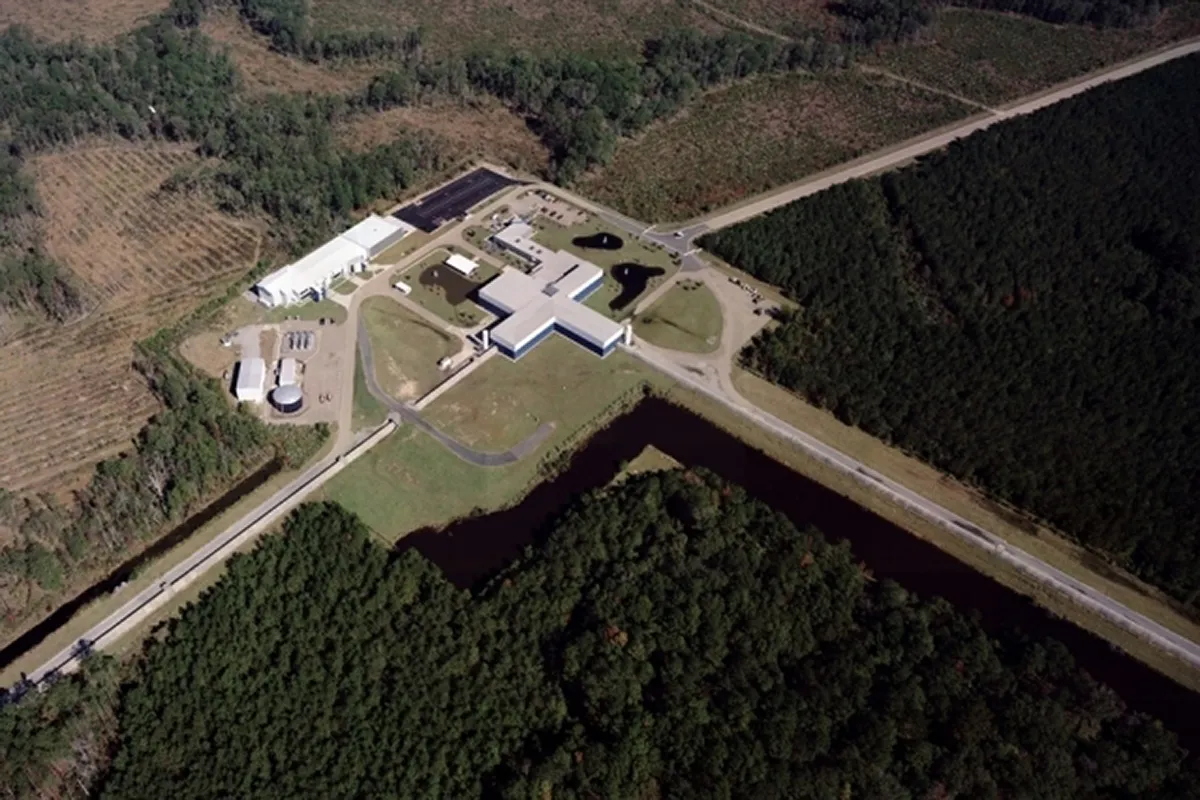
The difficulty in picking up gravitational waves
The changes being looked for are miniscule, the equivalent of measuring the distance to the Sun down to the width of a hydrogen atom.
To be sure of what they are seeing, several of these massive detectors need to be built to cross-check any signal between them.
This also allows scientists to learn where the waves have come from.
A single detector isn’t sensitive to direction, but with three, the location of the event that produced the wave can be pinpointed.
The other big problem is seismic noise.
Movements in the Earth from geological processes or even nearby traffic cause the ground to shake, masking the subtle changes.

A lot of sophisticated engineering has gone into reducing this, but so long as the detectors remain Earthbound, ground movement will always be a problem.
Scientists are looking skyward for the next generation of detectors.
The Laser Interferometer Space Antenna (LISA) will consist of three probes flying in formation through space, 5 million km from each other.
With a much longer distance to look for changes over, and no seismic noise to worry about, this space-based observatory will hopefully be able to pick up gravitational waves as they happen.

Why study gravitational waves?
Gravitational waves are vital to testing our knowledge of the Universe and understanding the rules that govern it.
Although we know a lot about what gravity does, we know little about the way it works.
Some 96% of all matter in the Universe isn’t thought to interact with light in any way, making it invisible.
Gravitational waves give us a new way of ‘seeing’ into the hidden dominion of dark energy and dark matter.
4 ways gravitational waves can occur
Inflation

A fraction of a second after the Big Bang, the Universe rapidly expanded.
This massive acceleration created huge gravitational waves that spanned the entire Universe.
Though these waves have since died away, they left their mark in the matter of the Universe, much like a wave on a beach leaves ripples behind on the sand.
Black hole collisions
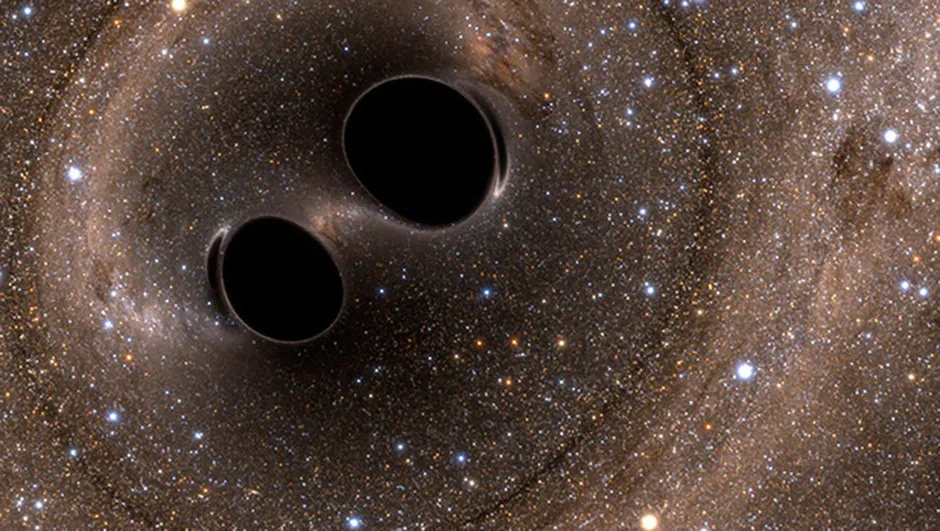
When two black holes orbit around each other they radiate an enormous amount of energy in the form of gravitational waves.
As they lose energy, the black holes begin to spiral in towards each other, eventually merging.
Many supercomputer simulations have been run to test how these mergers would appear to gravitational wave detectors.
Wobbling neutron stars
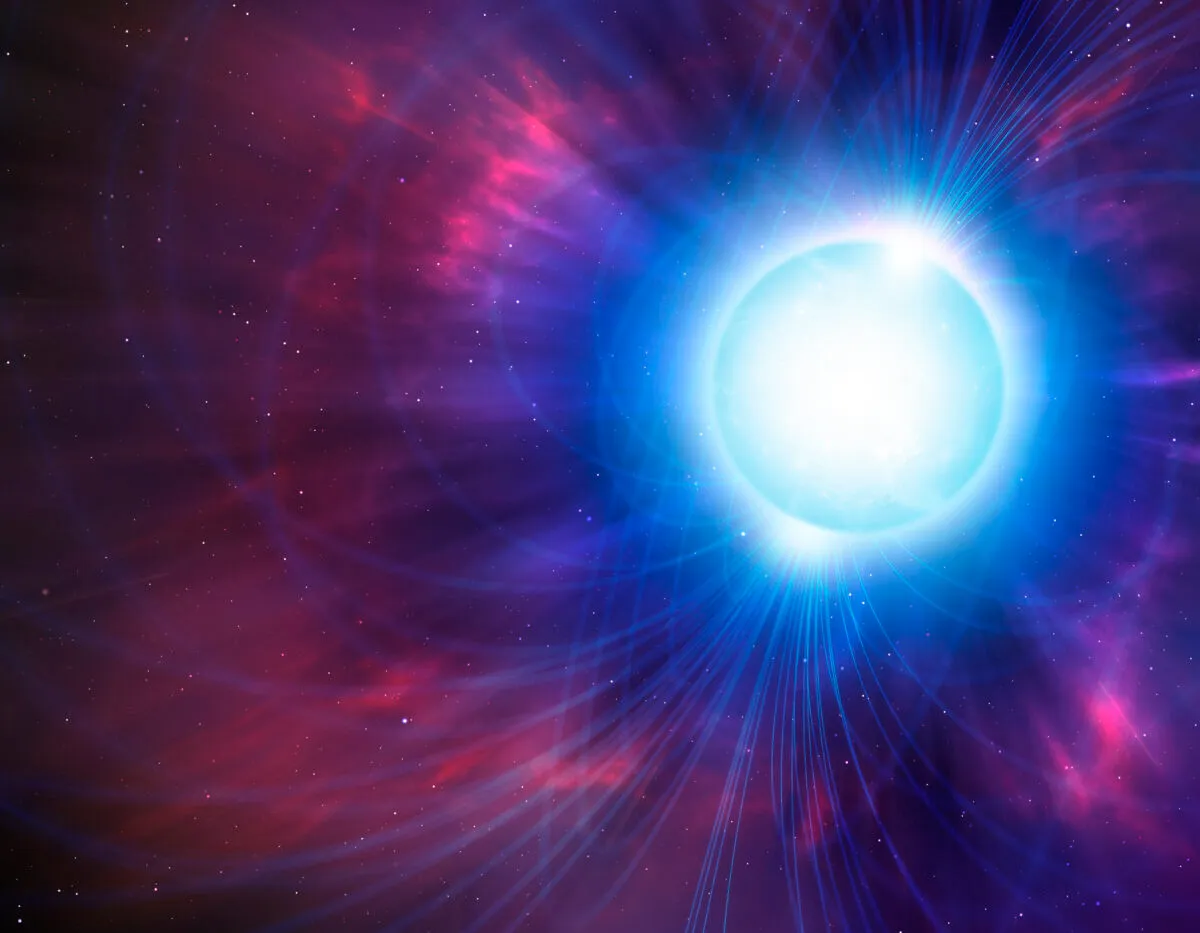
Neutron stars are the dense stellar cores left behind after some supernovae.
Most neutron stars spin rapidly; as they are so dense, any bumps on the surface of the star curve spacetime enough to produce gravitational waves.
Even if these ‘mountains’ can only reach a few centimetres in height before collapsing under their own weight.
Supernova
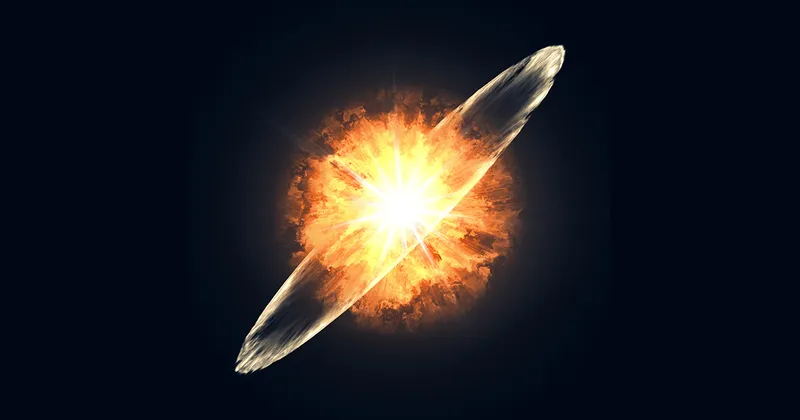
When a massive star goes supernova it collapses in seconds, sending out shockwaves through spacetime.
The waves produced are strong enough to be detected, but are still weaker than those produced by black holes.
A supernova would have to happen within our Galaxy for us to be able to detect the gravitational waves from it.
How interferometers work

Einstein never believed the tiny ripples could ever be measured and, to begin with, it appeared he was right.
When American physicist Joe Weber set up simple experiments to detect gravitational waves in the late 1960s and early 1970s, his results were unconvincing.
But scientists were certain that if gravitational waves did exist as Einstein predicted, they could be detected.
So they set out to build interferometers – huge, expensive detectors that measure the minute variations in the length of laser beams caused by a passing gravitational wave.

An interferometer splits a laser beam and sends it along two perpendicular arms of exactly the same length.
Mirrors at the ends of the arms reflect the beams back to a detector that picks up the pattern made by the beams’ lightwaves as they meet.
Provided both beams travel exactly the same distance, the patterns will match, cancelling each other out.
But they won’t if anything alters the distance one beam travels, such as a ripple in spacetime – a gravitational wave, in other words.
And by analysing the variations in the light patterns, scientists at laser interferometer observatories, such as LIGO, can determine how the gravitational wave was generated.
First gravitational wave detection

LIGO was inaugurated in 1999. It consists of two observatories, one in Hanford, in America’s Pacific Northwest and one near Livingston, Louisiana, some 3,000km to the southeast.
Detections are only taken seriously when both observatories see the same effect within 10 milliseconds of each other (gravitational waves travel at the speed of light).
In Europe, a similar but smaller detector called Virgo was built near Pisa, Italy.
Nothing definitive was detected at either facility during the first decade of the 21st century and so a programme of upgrades was undertaken to turn them into ‘Advanced’ sites with more powerful lasers, better vacuum systems and more stable mirror suspensions.
And then it happened. On Monday 14 September 2015, at 09:51 UT (10:51 BST), just four days before the Advanced LIGO was due to officially begin observations, a powerful gravitational wave (now known as GW150914) hit Earth from the south.
It passed through our planet, hitting LIGO Livingston seven milliseconds before arriving at LIGO Hanford. "We were very lucky," says Reitze. "Both detectors had already started operations."
Although rumours started to circulate, scientists managed to keep the news secret while they had analysed and confirmed the detection.
Finally, on 11 February 2016, the news broke.

What caused the first detected gravitational wave?
From the shape of the gravitational wave, scientists deduced that it had been caused by an orbiting pair of black holes, 29 and 36 times the mass of the Sun, spiralling into each other in a galaxy 1.5 billion lightyears away.
Just before they collided, they were moving at half the speed of light, completing 75 orbits per second.
Then, as they merged, the equivalent of three solar masses was converted into energy, leaving a 62-solar-mass black hole behind.
For a fraction of a second, the power emitted in the form of gravitational waves reached a value of 3.6x1040 gigawatts – about a hundred times the total power of all the stars in the observable Universe.
This article is an amalgamation of two articles that appeared in the May 2014 and May 2016 issues of BBC Sky at Night Magazine.