String theory posits that the particles we see are 1D strings of energy. Image Credit: Science Photo Library
General relativity was developed as an attempt to unify the forces of electromagnetism and gravity.
It was a task that obsessed Einstein for more than eight years as he sought to include gravity in his already successful special theory of relativity.
Before general relativity was laid down, Newton’s Laws of gravity had reigned for more than two centuries, but something was lacking.
The theory hinged on a mysterious and invisible force known as gravity that held the Universe together, but gave no real explanation as to what caused it or how it worked, only its effects.
As technology advanced, new precise measurements were beginning to show the flaws.
Observations didn’t match up to theory, and by the beginning of the 1900s people were looking for a new explanation of gravity.
It was these problems that Einstein created relativity to fix. But his theory was so strange that even after definitive proof had been provided it would take several years for general relativity to really gain a foothold.
Once it had, however, it completely changed the way that we looked at our Universe and now, 100 years later, it underpins nearly all modern physics.
General relativity states that everything that has a mass, from atoms to galaxies, sits within a four-dimensional spacetime that is bent and shaped by objects as they travel through it.
The larger the mass of an object, the more it distorts spacetime around it.
These disturbances in the fabric of spacetime then go on to affect everything that moves through it, including light.
This idea provided the foundation for our understanding of black holes, gravitational lensing and gravitational waves.
“General relativity is an extremely successful theory,” says Abhay Ashetekar, director of the Institute for Gravitation and the Cosmos at Pennsylvania State University.
“But it has limitations. At the very beginning of the Universe or inside a black hole, relativity predicts that physical limits become infinite and physics as we know it comes to an end.
This is showing that the theory has been pushed beyond its validity.
It’s a signal that a new theory is needed.”
Now attempts are being made to reconcile it with the other great theory of our Universe: quantum mechanics, the physics of nanoscopic scales where everything is made up of individual discrete units called quanta. But uniting these two conflicting systems is not straightforward.
Where gravity deals in absolutes, where every action causes a known reaction, quantum mechanics gives only the probablity of something happening. Trying to mesh them into a theory of ‘quantum gravity’ has met with little success.
The most well-known attempt to do so is string theory, which postulates that what we see as particles are actually vibrating one-dimensional strings of energy.
Other theories, such as quantum loop gravity, attempt to quantise gravity, pixellating the fabric of spacetime into individual quanta so that if you could look at it up close you would see structure that isn’t visible on a macroscopic scale. “
General relativity absorbs gravitational fields into the very geometry of spacetime,” says Ashetekar.
“If that’s true then the geometry of spacetime should be quantum mechanical. Spacetime itself should have fundamental building blocks. There should be atoms of geometry.”
Testing falters
Unfortunately, trying to observe these ‘atoms of geometry’ is currently beyond the realms of technological possibility.
This is one of the fundamental problems experienced by those investigating quantum gravity.
While it’s possible to produce elegant simulations and equations to describe the Universe, proving them is exceptionally difficult.
There are teams at work trying to pick out the traces of quantum mechanics left behind in the very early Universe by observing, for instance, the cosmic microwave background.
However, trying to test the theories directly requires a phenomenal amount of energy, far beyond what humankind is currently capable of producing.
But it is not uncommon for scientific theory to make predictions beyond the limits of what can be tested, only for technology to catch up later.
This, notes Martin Hendry, professor of gravitation and astrophysics at the University of Glasgow, was the case for another phenomenon predicted by general relativity: gravitational waves.
“Gravitational waves are produced by astrophysical sources in the Universe moving through spacetime, when objects such as black holes and neutron stars collide and combine together. Then they spread out like ripples in a pond.”
The search for these gravitational waves (or ‘gravy waves’ as they are known to those that study them) is a major focus of research today.
They were proposed soon after Einstein’s 1915 paper was published, but it has taken the intervening century for technology to catch up to the point that we might be able to find them.
Today’s detectors are huge, using lasers to accurately measure distances of several kilometres, searching for the tiny changes that would be caused by a gravitational wave passing through and stretching or shrinking spacetime.
It’s no easy task, as the changes being looked for are smaller than the size of an atom.
Luckily, two of the biggest detectors, LIGO and VIGRO, are in the process of being upgraded.

Perhaps then they will finally be able to record one; most likely caused by two massive bodies colliding.
“Up to now, these disturbances have been completely swamped by others due to all sorts of local noise sources,” says Hendry.
“But the prime candidate for astronomical signals that can be detected is the coalescence of compact binary systems.”
When dense sources spiral into each other and merge together, an enormous amount of gravitational energy is radiated off in the form of waves.
These may just be large enough to be detected here on Earth.
Though such waves have yet to be detected directly, there is already some indirect evidence for them.
“There is a neutron star binary system, the Hulse-Taylor binary, which is probably the most convincing piece of evidence we have that gravitational waves exist,” says Hendry.
“General relativity predicts that energy should radiate away in the form of gravitational waves, causing the orbits to shrink.
The change in the orbital parameters of that binary system agrees beautifully with the predictions of general relativity.”
Discarding the chaff
One of the biggest problems facing those running the detectors, however, is knowing what to look for.
“One of the interesting things about general relativity is that it’s non-linear, you can’t superimpose two gravitational fields,” says Harvey Reall, professor of theoretical physics at the University of Cambridge.
This means you cannot simply add two gravitational fields together, say that of the Sun and Earth, and find out exactly what the end result would be.
Though there are many good approximations for weak gravitational fields, when the field is strong – as it would be around a black hole – combining the effects of two is nearly impossible to do directly.
Instead, researchers had to run computer simulations to get an estimate.
“Researchers started simulations of merging black holes in the 1970s,” says Reall.
“They could do one orbit before the code crashed. But in 2005, two teams finally managed to crack the problem.
Now people routinely extract the gravitational waves emitted by black holes and neutron stars.”
Knowing what gravitational waves might get emitted makes the job of picking out the signal from general noise much easier.
With the next generation of detectors already being planned, it’s hoped that gravitational waves will be detected within the next few decades.
“Once we know how to look at the Universe using gravitational waves, the hope is we’ll see things that were completely undetected.
It’ll be a completely new way of doing astronomy,” says Reall.
Gravitational waves will open up a new way of studying the cosmos, as relativity gave us a new framework to think about the world around us.
One hundred years after Einstein first proposed his great theory, research teams all over the world are working with and developing his equations, coming up with questions no one had even thought to ask a century ago.
Hopefully by the time the next centenary comes around we’ll have at least some of the answers.
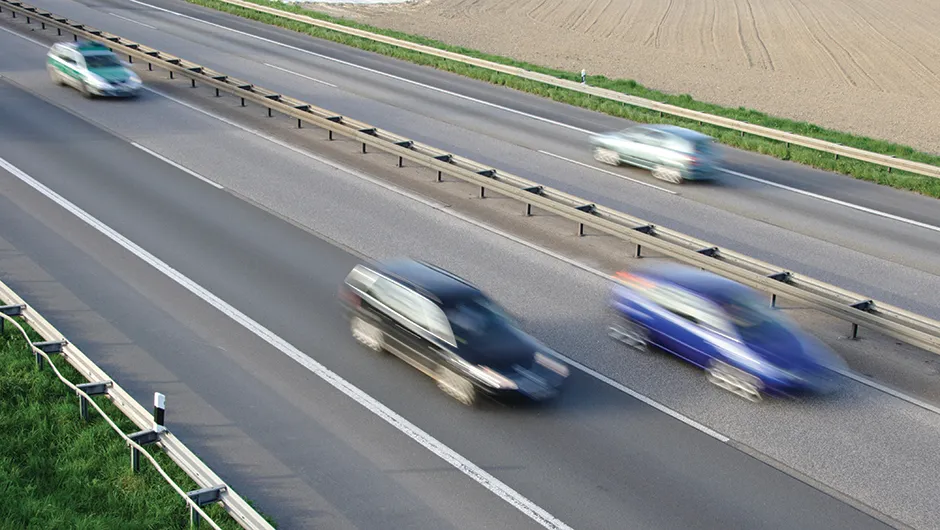
What’s special about relativity?
Ten years before his general theory, Einstein published a paper on special relativity.
This stated that no matter how fast you were travelling, the laws of physics remained the same within your own sphere of reference.
One of the key concepts was the universal speed limit of light.
Before Einstein it was assumed that the speed of light changed relative to the observer.
If you were moving relative to a light source, then this difference in speed would change the speed of light, in the same way that if you were driving on a motorway and overtook another vehicle, it would appear to be travelling slowly compared to you regardless of its actual speed.
What relativity states is that the speed of light remains fixed, no matter what the speed of the observer. Instead the fabric of spacetime changes to ensure the speed limit is kept.
The ‘special’ part about this initial theory was that it only considered what happens with regard to objects moving at high velocity.
When composing his general theory, Einstein took these ideas and played them out across the entire Universe, such as when objects are accelerating.
This was important as he could then substitute out the effects of acceleration for those caused by gravity, giving him the framework that would go on to form the basis of most modern cosmology.
This article originally appeared in the June 2015 issue of BBC Sky at Night Magazine.