Sometimes called ‘the afterglow of creation’, the cosmic microwave background is considered one of the most convincing pieces of evidence for the Big Bang theory.
But how is it that we are still observing this ancient light?
As we known, space is expanding. Since the amount of matter in the Universe remains fairly constant, the increasing volume means that the average density of cosmic matter is decreasing over time.
That means the density of the Universe must have been much higher in the distant past.
Right after the Big Bang, before there were any galaxies, stars or planets, elementary particles were packed almost shoulder to shoulder, and the corresponding temperature was unimaginably high.
This hot, dense plasma produced energetic radiation.

However, individual light particles – photons – have trouble traversing such plasma-filled environments, as they are continuously absorbed (and re-emitted) by charged particles like electrons.
In other words: the hot, early Universe was opaque.
As space expanded, it cooled, and when temperatures dropped below around 2,700˚ Celsius (much cooler than the surface of the Sun), electrons could combine with protons to form electrically neutral hydrogen atoms.
From then on, photons could freely stream through space, in all possible directions.
The Universe became transparent. This happened some 380,000 years after the Big Bang.
Incidentally, the average density of the Universe at that time was already extremely low: just some 1,000 atoms per cubic centimetre.
The radiation that was finally ‘released’ when the Universe was 380,000 years old is called the cosmic microwave background (CMB).
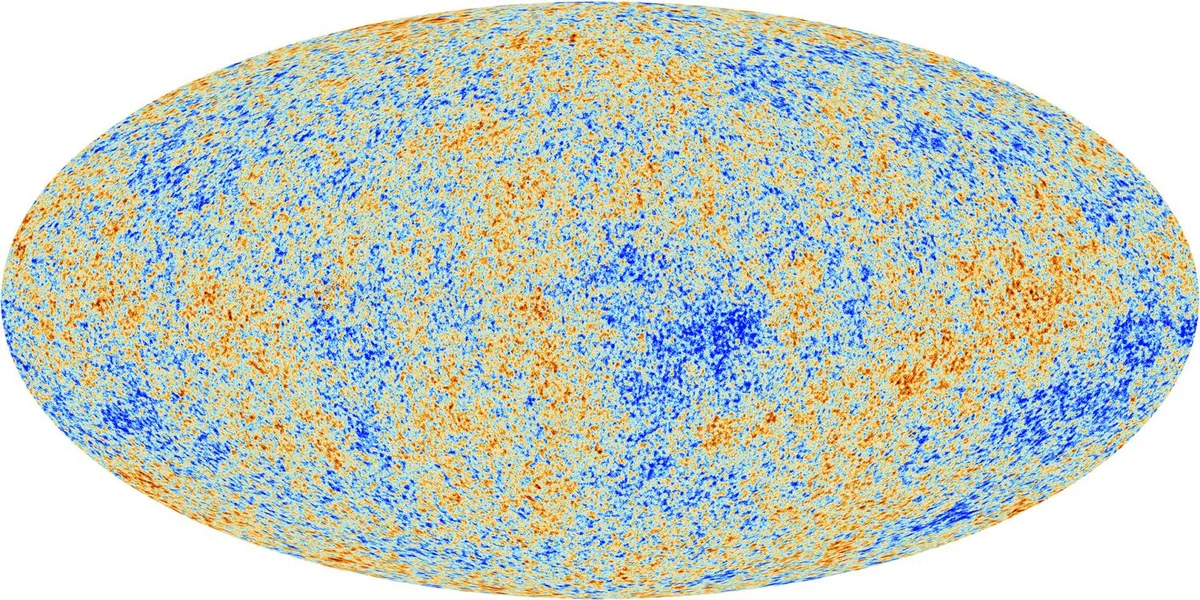
Although this radiation was originally emitted at optical wavelengths, it has travelled through expanding space for almost 13.8 billion years and as a result the waves got stretched all the way into the microwave part of the electromagnetic spectrum.
But if this radiation was emitted at more or less one single moment in time, how is it that we are still able to keep observing it?
And why do astronomers see it all over the sky? Shouldn’t the CMB have passed us long ago as a brief flash of light, coming from one particular direction?
Well, no. Remember that the Big Bang was not some fireworks explosion taking place at a particular location in empty space.
Instead, all of the Universe (including the part that we currently inhabit) was once in a state of extremely high density and temperature.
The primordial radiation emitted by our part of space some 380,000 years after the Big Bang has long disappeared into the distance.
But photons emitted by other, very remote parts of the Universe are only now arriving at Earth.
Cosmic microwave background explained simply

Imagine you are on a large city square filled with people, and let’s assume (for the sake of convenience) that the speed of sound is just one metre per second – much lower than its true value.
If everyone is asked to shout ‘Boo!’ at exactly 12:00:00, your own shout will die out pretty soon.
But 10 seconds later, at 12:00:10, you will hear the shouting from people who are 10 metres away.
At 12:01:00, the shouts from people at 60 metres distance will hit your ear drums.
Even at 12:10:00, 10 minutes after everyone produced their instantaneous ‘Boo!’, you receive the (softer) sound from a distance of 600 metres.
The same is true for the cosmic microwave background.
It was ‘emitted’ everywhere in space at more or less a single moment in time, but since it travels at a finite speed (the speed of light), we keep receiving the signal from ever-further regions of the Universe.
Today, 13.8 billion years after it began its journey, it arrives on Earth from parts that are now some 45 billion lightyears away – our cosmological horizon.
Little wonder that it’s such a faint signal!
What can the Big Bang afterglow teach us about the Universe?
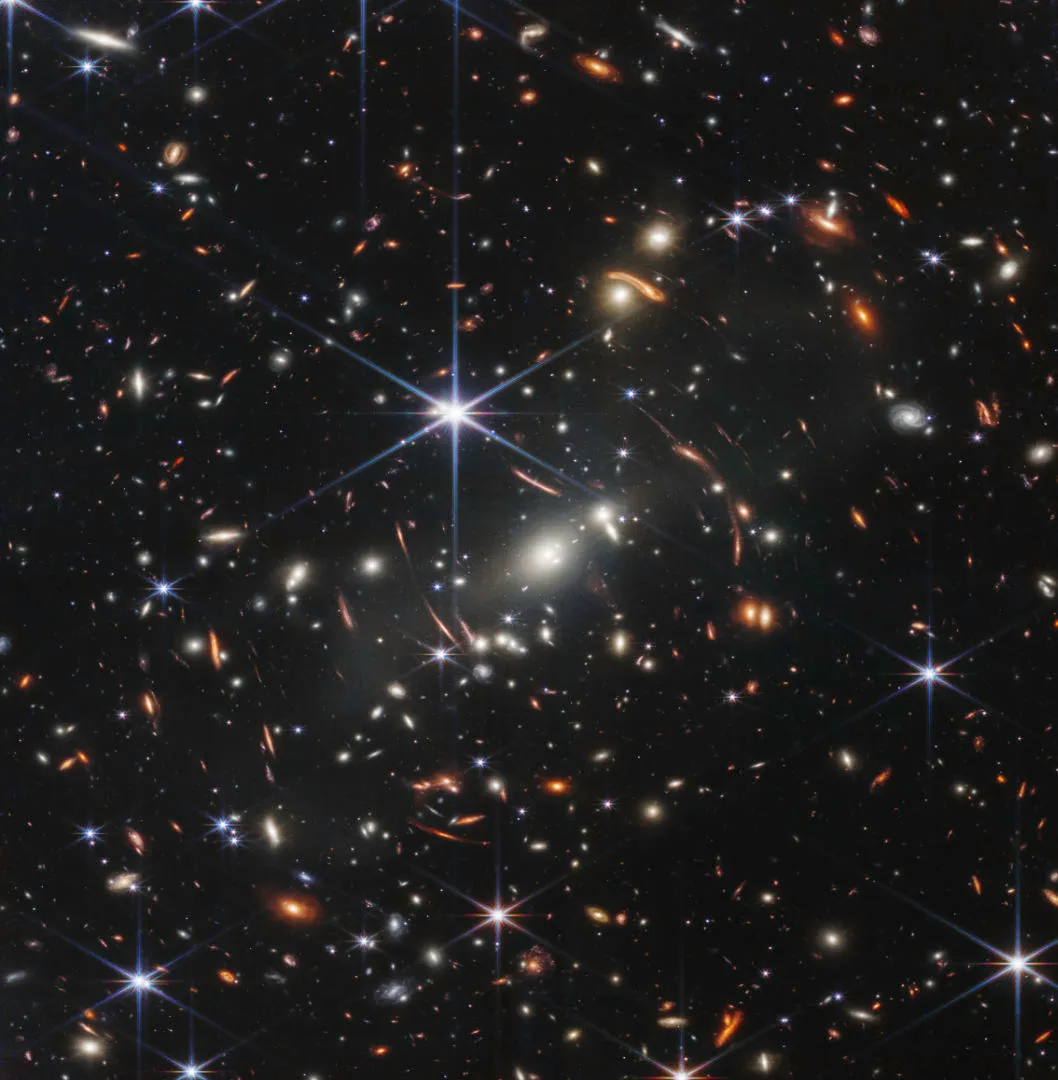
The cosmic microwave background was discovered in 1964 and studied in incredible detail by the European Planck mission.
It turns out not to be completely homogeneous: there are minute temperature variations in the CMB we see today, created by small density fluctuations when the Universe was around 380,000 years old.
Over the course of its 13.8-billion-year history, these density fluctuations have given rise to the rather clumpy large-scale structure of the present Universe, with galaxies, clusters, and superclusters.
This growth process was governed by the global properties of the cosmos, such as the abundance of ‘normal’ and dark matter, the expansion rate and the amount of dark energy.
By meticulously comparing the Planck data with observations of today’s Universe, scientists have been able to quantify these cosmological parameters to a high accuracy, even though the true nature of dark matter and dark energy is still a mystery.
This guide originally appeared in the May 2023 issue of BBC Sky at Night Magazine.