The inside of a star is a noisy place to be, as many stars hum songs to themselves. We can’t hear these songs directly because the sound waves cannot escape the star, but they do create a visible effect on the surface.
Sound waves continuously bouncing around inside a star cause it to swell and contract, and these movements cause changes in the temperature at the surface, which can be detected as variations in the brightness of a star.
All stars have a pattern of brightness that changes over time, known as a light curve, but if there are numerous sound waves at once this pattern can become crowded and difficult to analyse.
Read more about stars:
A mathematical technique known as a Fourier transform can be used to pluck the individual frequencies out of the light curve.
By measuring characteristics such as the spacing between frequencies, it is possible to learn a lot about the star such as its mass, size, and age.

The Kepler Space Telescope stared at the same patch of sky for four years, which is crucial for detecting some of the ‘quieter’ sound waves.
While the primary aim of the Kepler mission was to search for exoplanets, the lengthy observations needed to find planets are also perfect for picking out the subtle frequencies in hundreds of pulsating Sun-like stars.
“This is opening the possibility to perform detailed studies of the evolution and internal structure of stars like the Sun”, says Bill Chaplin of the University of Birmingham.
These pulsations are much easier to detect in red giant stars than those less advanced in the stellar life cycle because the periods of the sound waves in red giants are much longer.
Younger stars have periods of only a few minutes, whereas a sound wave in a red giant will take hours to oscillate back and forth.
The interiors of red giants change dramatically as they evolve further. Having exhausted their supply of hydrogen in the core, red giants burn hydrogen in a shell around a dead core.
The core eventually reignites, this time burning helium. In both cases the red giant looks the same on the surface – but Kepler showed that the asteroseismic signatures are very different.
Distinguishing the two populations of red giants is a leap forward in our understanding of stellar evolution.
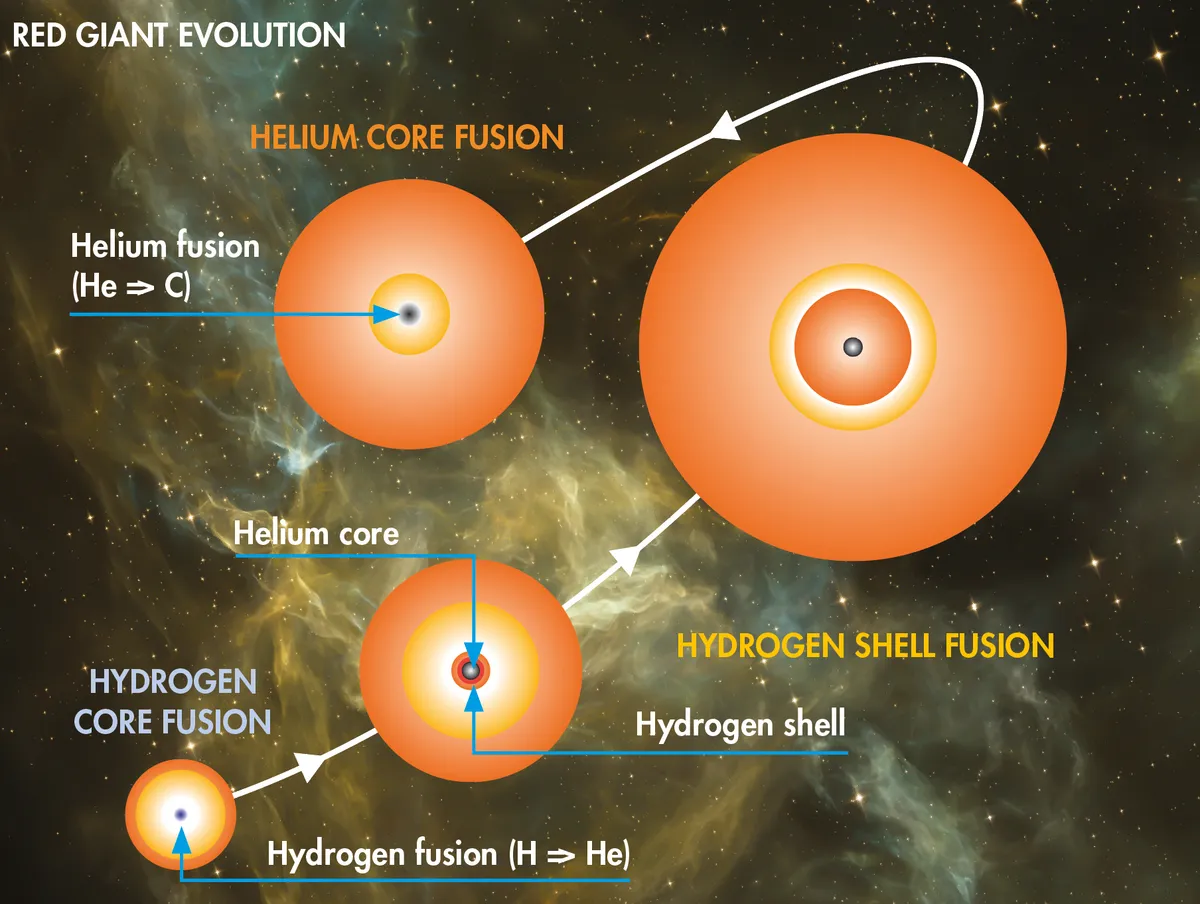
Stellar insights
Delta Scuti variables and Gamma Doradus variables are types of main sequence star that have distinctive pulsation periods.
Both classes are hotter than the Sun, and typically Delta Scuti stars have higher temperatures than Gamma Doradus stars.
The different temperature ranges mean that these stars sit within their own region on the Hertzsprung-Russell diagram (see below), which is widely used to compare the temperature and brightness of stars in order to track their evolution.
Theory predicts that there should be a handful of ‘hybrid’ stars in the overlap area between the Delta Scuti and Gamma Doradus classes, and that these hybrids will show both types of pulsations.
It therefore came as a surprise when Kepler revealed hundreds of hybrid stars that were littered across both the Delta Scuti and Gamma Doradus regions of the Hertzsprung-Russell diagram.
In addition, some stars that should show pulsations remain mysteriously quiet.
Another curious discovery made by Kepler was unusual double periods in RR Lyrae variables.
These typically have pulsation periods of around half a day, but some of them also exhibit a longer period where the overall shape of the light curve changes on timescales between tens to hundreds of days, which is known as the Blazhko effect.
Kepler data revealed that the sound waves in some of these stars can vary on short timescales or on long timescales, and alternate between the two, an effect known as ‘period doubling’.

Period doubling is known to occur in other types of variable stars, but was never seen in RR Lyrae stars prior to Kepler.
Strangely, period doubling only occurs in stars that also exhibit the Blazhko effect, indicating a connection between the two types of periods.
The Kepler mission played a vital role in these discoveries and the field of asteroseismology, but in 2013 two of the telescope’s reaction wheels failed and it looked like the mission was at an end.
However, it was rebooted as K2, which observed different fields for around 80 days each. Asteroseismology needs lengthy observations for the utmost precision. The K2 Galactic Archaeology Programme surveyed red giants over a large proportion of the Galaxy.
“In essence what we do is to use the stars as probes of the Galaxy’s structure and we use the stellar ages as the clock to obtain a picture of how the Milky Way evolved over its 13 billion-year history,” explains Dennis Stello of the University of Sydney, who analyses K2 data as part of his work in the university's Stellar Oscillations Group.
Stars like our Sun can be studied, and the necessity of observing brighter stars means that information will be available on those stars from other ground-based observational methods, allowing for better characterisation.
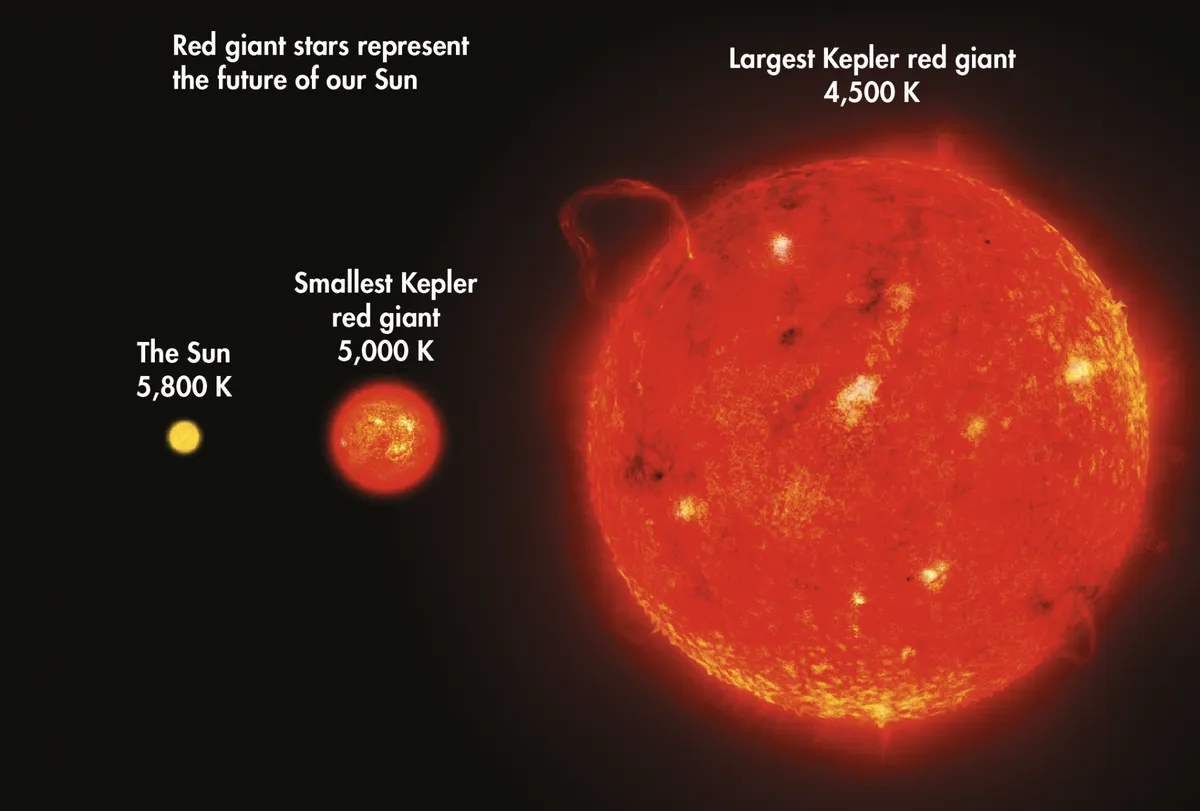
Spinning faster on the inside
Main sequence stars have two different types of sound waves – those that reverberate in the outer layers of the star, and those that are restricted to the core of the star.
Analysing core sound waves would reveal the innermost workings of a star, but it is currently impossible to detect these waves in stars like our Sun.
As a main sequence star evolves into a red giant, the changing density in the core allows the sound waves to drift upwards and interact with the waves in the outer layers, making them visible to astronomers.
Normally, a sound wave that has been extracted from the light curve will show a single peak at a certain frequency.
However, if the star is rotating then this single peak can get split into several peaks. By measuring the splitting of the frequency of the wave, it is possible to measure how fast the star is spinning.
With the Kepler red giant data, rotation splitting can be measured for both the core and for the surface.
Comparing the two data sets has shown that some red giant stars rotate much faster on the inside.

Stars like our Sun
Studying oscillations in our Sun, known as helioseismology, began in 1962, and having decades of data on the Sun has revealed much about our star.
For example, some pulsation frequencies vary with the 11-year activity cycle.
Helioseismology has paved the way for asteroseismology. While the disc of the Sun can be resolved, observing the Sun as if it were a distant point source has ensured that the knowledge gained through helioseismology can be exploited when looking at other Sun-like stars.
The Kepler mission measured oscillations in over 500 Sun-like stars, deciphering parameters such as mass, radius and age.
Before Kepler, only around 20 stars had measured pulsations. The stellar parameters from asteroseismology are generally measured to unprecedented precision, which is of particular importance for stars that host exoplanets, and thus tie in nicely with the main objective of the Kepler mission.
Without knowing the details of the host star, it is impossible to pinpoint the planet’s properties. For instance, knowing the mass and radius of the planet will help to reveal if it is comprised of dense iron or porous rock.
Dr Amanda Doyle is an astrophysicist based at the University of Warwick.