What would you think if you were told there was an equation for discovering the likelihood of life beyond Earth? Ever heard of the Drake Equation?
Some years ago, researchers asked 2,000 people what scientific question they would most like answered. Top of the list was: are we alone in the Universe?
I was surprised (and quite heartened) by the fact that so many people thought this question was important, as it has always intrigued me too.
But are we to getting any closer to an answer to that question: will we ever find life beyond Earth?
In 1961 American scientist Frank Drake tried to quantify the problem by looking at probabilities, laying down the parameters that would affect the numbers of active, communicative civilisations in our Galaxy.

He came up with a calculation that has become known as the Drake Equation. The Drake Equation can be expressed thus:
N = R* x fp x fg x ne x fl x fi x fc x L
What the letters in the Drake Equation mean
R* = the rate of yearly star formation in the Galaxy
fp= the fraction of stars with planets orbiting them
fg= the fraction of stars that could support habitable planets
ne = the number of planets that can potentially support life (per star with planets)
fl = the fraction of planets that actually develop life at some point
fc= the fraction of civilisations that emit detectable signs of their presence
L= the average length that a civilisation exists
Finding values for the Drake Equation

OK, so we have Drake's famous equation, but how well can we actually apply values to the various parts of the formula?
When Drake first considered the equation some 53 years ago, only the first term, R* – the rate of yearly star formation in the Galaxy - how many stars are born - was known with any confidence.
But with increasingly sophisticated equipment, we’ve gleaned more and more information from our observations.
Our estimate of fp, the fraction of stars with planets orbiting them, has greatly improved since the discovery of the first exoplanet in 1992.
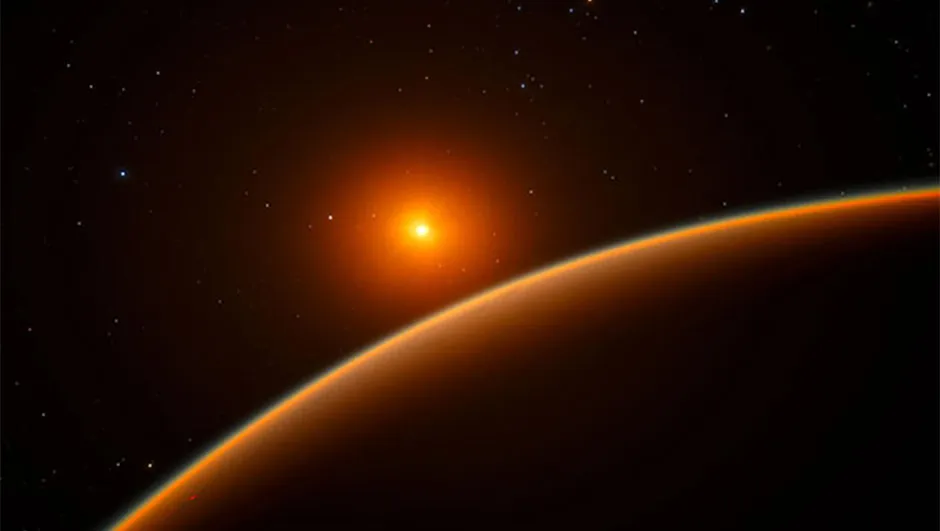
Values for fg, the fraction of stars that could support habitable planets, is also better understood today, although our definition of what makes a planet habitable is limited to the one example we have – Earth – and so hinges on the availability of liquid water.
The next parameter, ne, the number of planets that can potentially support life (per star with planets), has driven our search for Earth-like planets rather than the larger, easier to detect Jupiter-like worlds that earlier searches revealed.
At this point we reach a bit of a boundary, and we have to resort to guestimation rather than estimation to fill out the rest of the parameters.
We are on the cusp of being able to measure the initial signs of fl, the fraction of planets that actually develop life at some point, using spectral analysis.
Life as we know it leaves chemical signatures in a planet’s atmosphere - biosignatures - which could be detected by analysing light absorption through it.
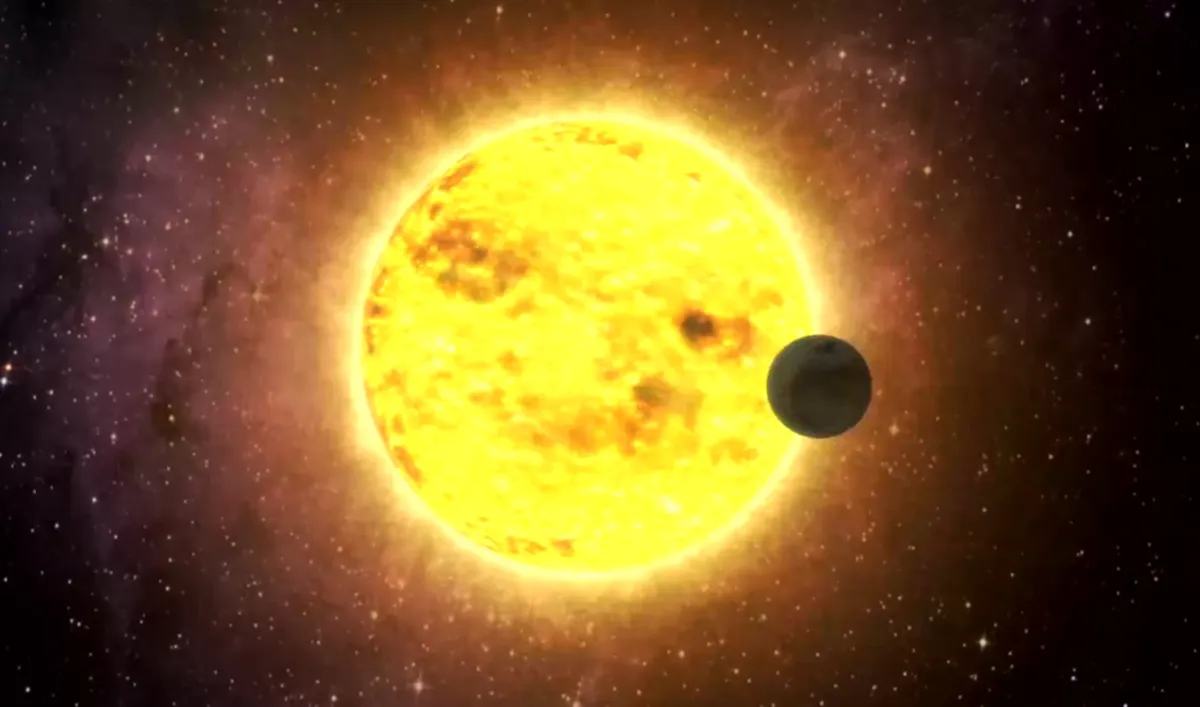
It may even be possible to get a feel for fi, the fraction of planets that will develop intelligent life, using a similar method.
Some chemicals do not occur naturally, so if we detect them on an exoplanet it is likely that its population has made technological advances.
The final two terms prove to be even harder: fc, the fraction of civilisations that emit detectable signs of their presence, and L, the average length that a civilisation exists, are very hard to judge when you have a sample of just one, us.
As a result of the nebulous nature of these latter terms, estimates for the value of N – the number of civilisations in our Galaxy which we may be able to communicate with – can vary by more than 10 orders of magnitude.
This could be seen as a problem, but that might perhaps be missing the point of the Drake Equation, as it should not be seen as less of an estimating tool and more of a blueprint for how to go about searching for life in the Galaxy.
This article originally appeared in the October 2014 issue of BBC Sky at Night Magazine.