Around 13.8 billion years ago, a single, infinitely dense point suddenly expanded faster than the speed of light, producing a superheated fog of radiation and particles.
This was the birth of the Universe in the Big Bang – the ultimate cataclysmic event.
The Big Bang is our best model to explain the Universe and the laws of physics as we understand them, including how space, time and matter came to exist.
The more we study the Big Bang, the more we can learn about how the cosmos began and evolved into the Universe we see today.

By studying it, we’ve learned the expansion of the Universe is accelerating.
Meanwhile, fluctuations in the cosmic microwave background (the remnant light after the Big Bang) showed us dark matter and dark energy together account for an astonishing 95% of the total content of the cosmos.
But this isn’t the only cataclysmic scenario astronomers use to learn about the Universe.
From exploding supernovae to the violent mergers of supermassive black holes, dramatic events are happening across the cosmos all the time, each releasing a huge amount of energy.
Whenever one is spotted, scientists race to study them. But what is it that we love so much about big bangs?
Supernovae

Perhaps the best-known cosmic explosion is the supernova, when a massive star runs out of fuel
and explodes in a sudden brilliant flash of light, billions of times brighter than our Sun.
They are short-lived, and over a few weeks or months the light fades.
What remains is a cloud of hot gas expanding around a neutron star, a stellar core so unimaginably dense even a teaspoon of it weighs around a billion tonnes (2.2 trillion pounds).
In some cases, if the star was extremely massive before it exploded, it forms a black hole.

Supernovae interest astronomers for several reasons.
They happen fast, which is convenient.
Most cosmic processes last for millions or even billions of years and we have to piece together what happens during most of a star’s lifespan by observing similar stars at different stages of their lifecycle.
A supernova, though, we can watch from start to finish.
They’re also exceptionally bright, often outshining their entire galaxy, meaning they can be seen billions of lightyears away, so observing them helps us to see the large-scale structure of the Universe.

Type Ia supernovae are particularly useful in this regard.
These are caused when a white dwarf star in a binary system acquires so much material from its companion it reaches a critical mass – equivalent to 1.4 solar masses – at which point it collapses and then explodes.
Because they all explode at about the same mass, the amount of light released is very consistent.
Astronomers use them as ‘standard candles’ to measure cosmic distances accurately – if we observe two Type Ia supernovae and one appears dimmer, we know it must be further away.
Fast radio bursts
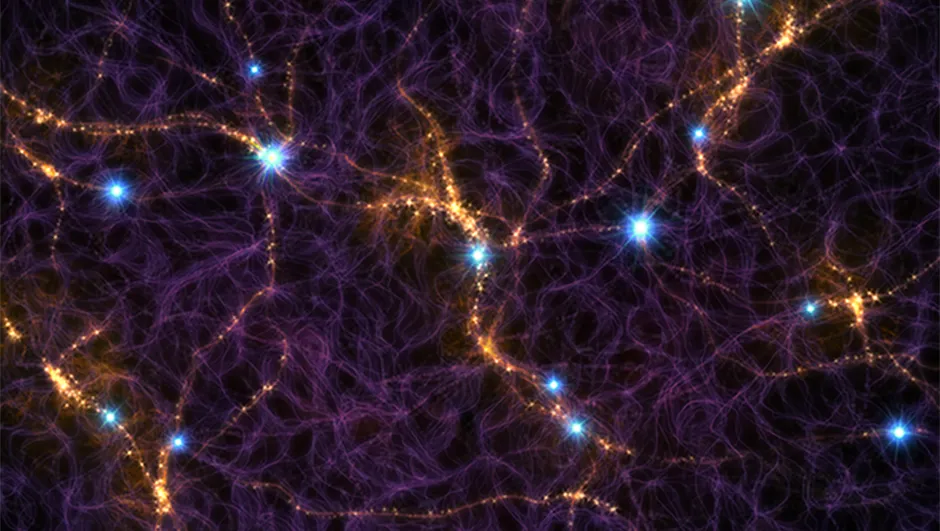
While supernovae have been observed since ancient times, a recently discovered variety of high-energy event is fast radio bursts.
Though we’ve only known about them since 2007, hundreds have been discovered.
They are extremely short-lived radio pulses, normally lasting a fraction of a second, and are incredibly powerful – we estimate that one fast radio burst can generate as much energy as our Sun does in a year.
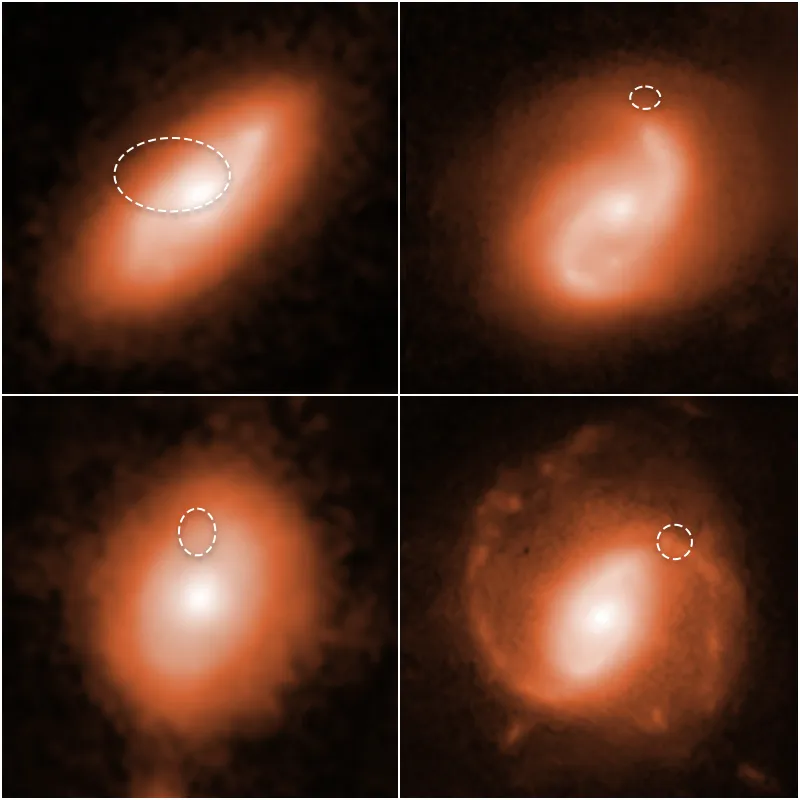
What causes them is still a mystery, but they are probably associated with dense objects like white dwarfs, neutron stars and black holes.
Some have even been detected emanating from areas where we know the supermassive black holes of colliding galaxies are merging.
Most of these bursts are transient one-off events, making them challenging to study – by the time you realise you’ve seen one, it’s already over.
But some fast radio bursts are repeating, opening up the possibility of observing them over time.
What triggers these repetitive bursts? We aren’t sure.

One idea is that repeaters are produced by a specific type of neutron star called a magnetar, which has an astonishingly powerful magnetic field.
The theory is this field stresses the magnetar’s crust, causing starquakes and huge flares of radiation.
Repeating fast radio bursts can act like a cosmic radar beacon, as their signals carry a signature of the material the burst travelled through.
This makes them an exciting new tool to quantify the amount of matter between galaxies and to study the 3D clustering of matter.
By allowing us to test and improve our cosmological models, they may even help us to resolve the Hubble tension and explain why the observed expansion of the Universe doesn’t match theoretical predictions.
Gamma-ray bursts
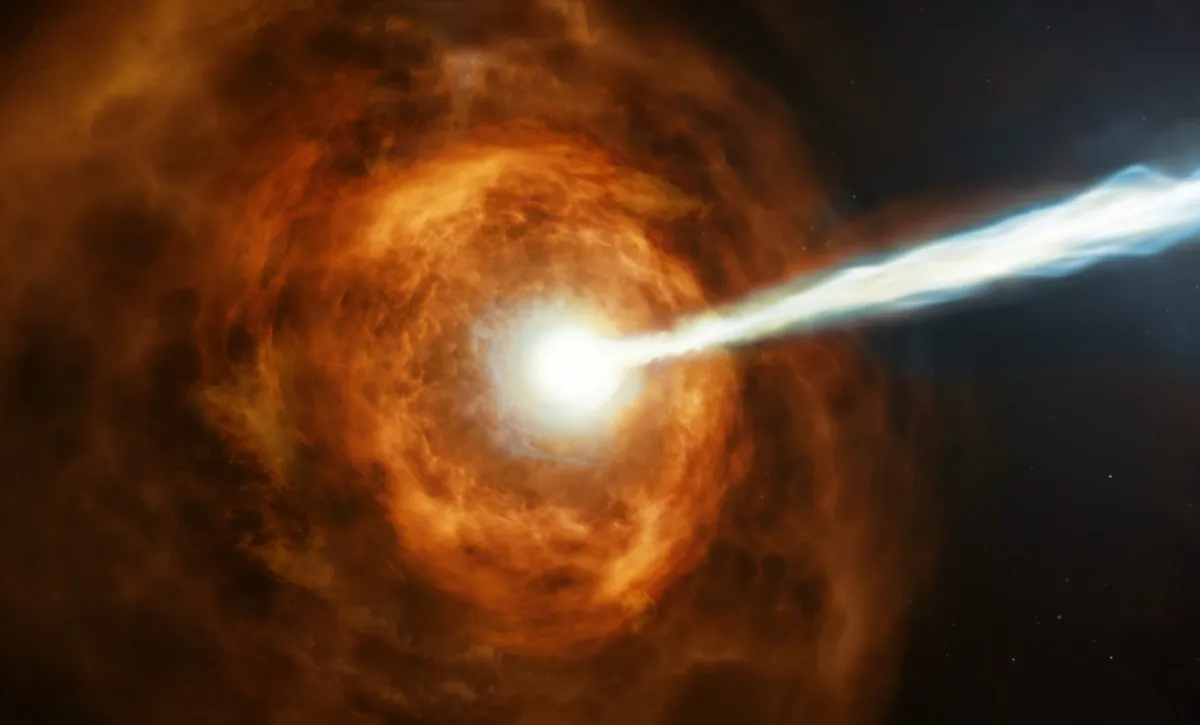
In terms of high-energy events, however, both supernovae and fast radio bursts pale in comparison to gamma-ray bursts.
These are the most powerful explosions we know of in the Universe since the Big Bang, generated when spectacularly violent events take place.
A typical gamma-ray burst releases more energy in 10 seconds than our Sun will during its entire 10-billion-year lifespan.
Some are short gamma-ray bursts, lasting milliseconds to two seconds, thought to be released when two neutron stars, or a neutron star and a black hole, merge to produce a new black hole.
By contrast, long gamma-ray bursts, lasting from two seconds to several minutes, are thought to be released when some giant stars go supernova, forming a black hole.

In 2022, an exceptionally bright gamma-ray burst lasting 10 minutes was discovered emanating from a supernova 2.4 billion lightyears away, which was so powerful it affected Earth’s atmosphere.
A gamma-ray burst this energetic and close to home is unusual, occurring perhaps once in 10,000 years.
Certainly, it was the most energetic burst we’ve observed and it is now referred to as the BOAT (Biggest Of All Time).
In both cases, the new baby black hole emits jets of energetic particles which produce bursts of gamma rays as they travel through the interstellar medium.

Astronomers can observe these incredibly powerful gamma-ray bursts over extreme distances.
As light takes so long to travel the enormous distances of the Universe, astronomical distance is proportional to time, allowing us to study the Universe as it was many billions of years ago.
This means studying gamma-ray bursts gives us fresh insights into the early Universe, including the dramatic births and deaths of early massive stars, and the formation of some of the first black holes.
Colliding black holes

It’s just as well that black holes are involved in so many of cataclysmic events, as they act as cosmic laboratories.
Black holes are so dense, their gravity so strong, that physics as we know it begins to break down inside them – they represent the very limits of our knowledge.
Studying them takes us one step further to understanding fundamental physics on the largest and smallest scales, testing what we know about both the general theory of relativity and quantum physics.
The challenge is that black holes themselves emit no light of any kind – we can only detect them indirectly by observing their effect on surrounding objects.
But one of the best opportunities to study them occurs when two collide and merge.
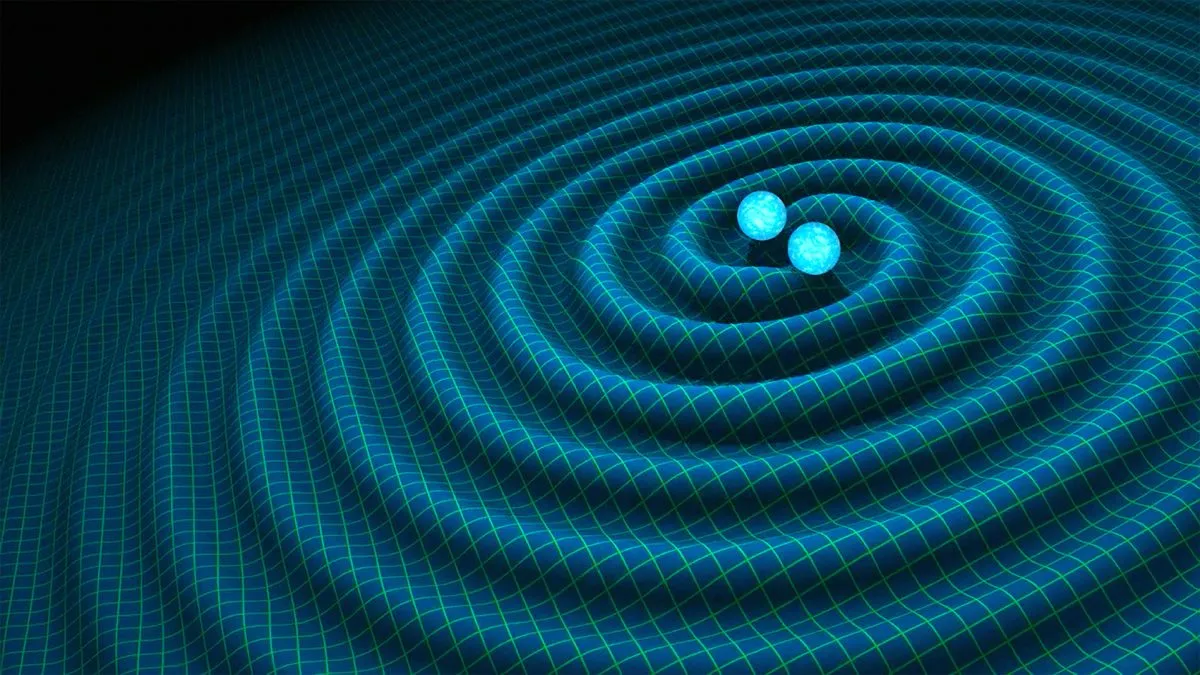
This truly cataclysmic event produces gravitational waves – ripples in space-time that race out across space, compressing and stretching anything they encounter.
These gravitational waves can tell us about the black holes’ size, how far away they were and how fast they were travelling when they collided.
Combining these measurements with more traditional electromagnetic observations – a technique known as multi-messenger science – tells us even more.
Supernovae and the mergers of dense and compact objects like black holes and neutron stars are some of the most extreme phenomena in the Universe today.
These dramatic and destructive events unfolding across the Universe on a colossal scale have much to tell us.
We will continue to learn as increasingly sophisticated observatories advance our ability to detect electromagnetic radiation and gravitational waves, and to study the cataclysmic ‘big bangs’ that release them.
This article appeared in the November 2024 issue of BBC Sky at Night Magazine