Infrared astronomy has become much better understood by non-astronomers in recent years, thanks in part to the incredible image and discoveries of the James Webb Space Telescope.
But why is infrared so good for observing the Universe, and why do astronomers choose to observe a galaxy or star-forming region in infrared rather than optical light?

Infrared is a form of electromagnetic radiation exactly like visible light, but with a longer wavelength.
The infrared waveband extends from 0.8 to 1,000 microns.
In astronomy, infrared enables us to view elements of the Universe that are invisible to the human eye.
Most of the infrared radiation from the night sky is absorbed by molecules of water and carbon dioxide in the Earth’s atmosphere, though there are a few atmospheric ‘windows’, which allow observations by telescopes on the ground.
It is only from space that we can see the full splendour of the infrared sky.

Infrared was discovered by astronomer William Herschel in 1800 when he split sunlight using a prism and measured the temperature beyond the red end of the spectrum.
We experience infrared when we feel the radiant heat from a fire or from human bodies, which we cannot see.
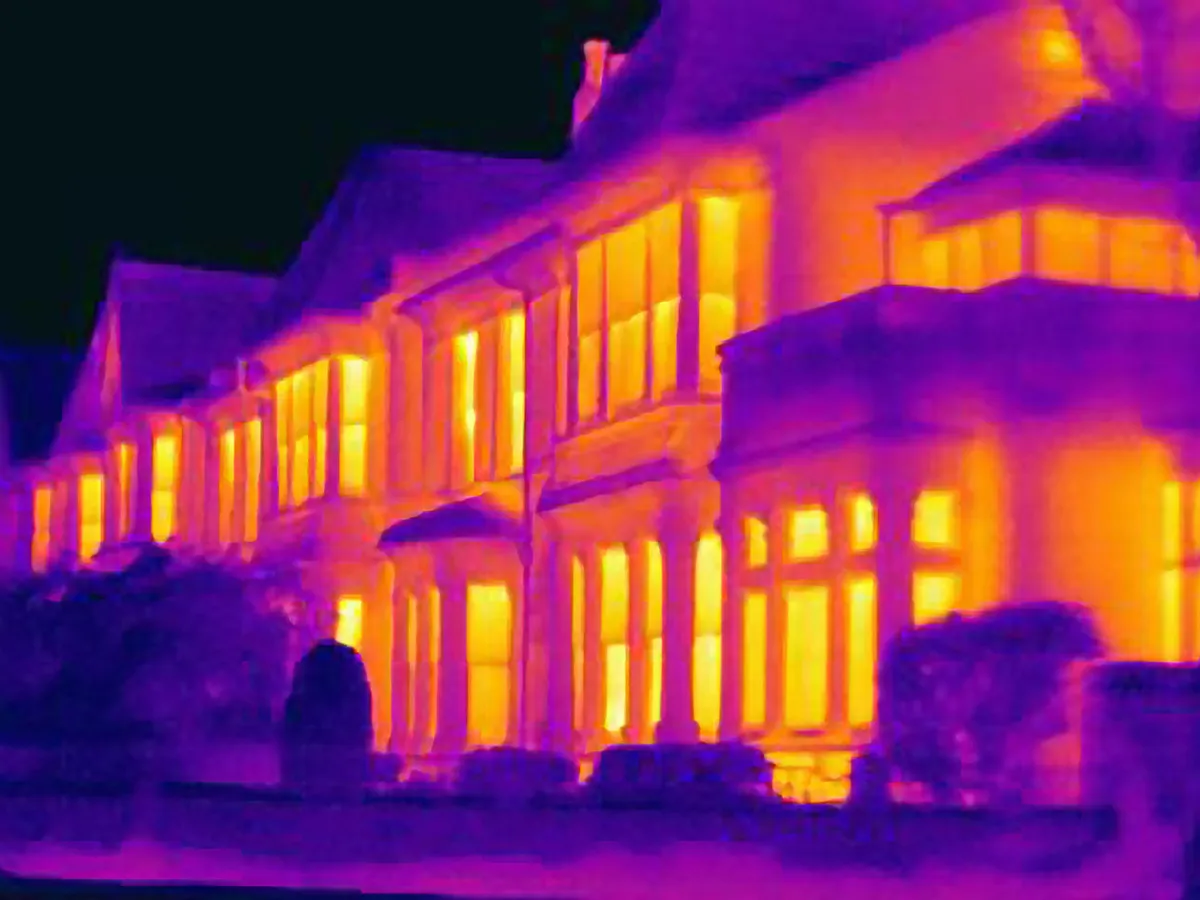
In astronomy, the importance of infrared wavelengths is their capacity to draw back the veil of obscuring dust that hides so much of the starlight of the Universe.
As much as two thirds of all light emitted by stars is absorbed by interstellar dust and re-emitted at infrared wavelengths.
Infrared astronomy and star formation

Only in the infrared part of the spectrum can we really assess how much star formation is actually taking place in galaxies, and how this changes with time.
Regions in our own Milky Way galaxy and others where new stars form are completely shrouded by dust, making infrared surveys crucial in trying to understand not just the origins of stars but of planetary systems too.
These small, sub-micron-sized dust grains are where most of the elements made in stars are stored.

The atoms of our bodies: carbon, nitrogen, oxygen and so on, were once locked up in dust grains between the stars.
Infrared observations help us to understand the composition of these grains and hence the chemical evolution of interstellar material.
They also help us to find out the physical conditions, density and temperature of the gas in regions where stars are forming.
In our Galaxy, the fraction of starlight absorbed by dust is about 30%, but when galaxies are younger this fraction is much higher.
Newly-formed stars are, in fact, totally shrouded by dust. So we can only trace out the history of star formation in the Universe by surveying in the infrared.
Using infrared to search for exoplanets
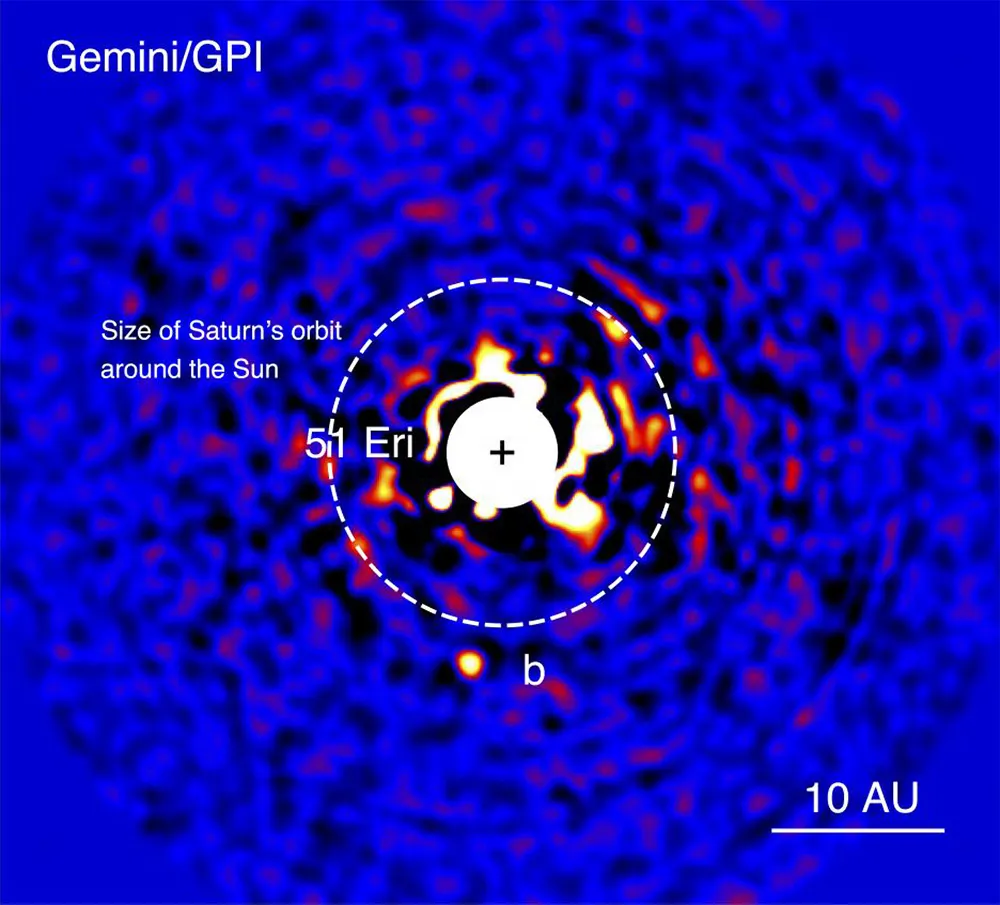
The infrared is also the ideal wavelength band to search for direct evidence of giant exoplanets and brown dwarfs – objects that are not quite massive enough to ignite nuclear burning and turn into stars.
The Infrared Astronomical telescope, IRAS, found several examples of stars surrounded by debris discs that are believed to be potential planetary systems in the making.
On the larger scale, the Japanese Akari mission searched for infrared monsters – galaxies hundreds of times more luminous than our Milky Way, which emit 99% of their output as infrared radiation.

Large-scale surveys can detect star-forming galaxies back to the first billion years of the Universe.
A second factor that drives us towards infrared in astronomy is the cosmological redshift.
The infrared Spitzer Space Telescope found galaxies with redshifts greater than six, which emit no optical light at all. Their redshifted starlight is detectable only in the infrared.
It is for this reason that the successor to the Hubble Space Telescope, the James Webb Space Telescope (JWST), is primarily an infrared instrument.
For more on this, read our guide on how Webb observes exoplanets and how Webb observes galaxies.

A history of infrared astronomy
The James Webb Space Telescope is the latest in a long line of infrared space telescopes.
The first, IRAS, surveyed the whole sky at wavelengths of 12 to 100 microns in 1983.
IRAS made discoveries in many different areas of astrophysics:
- Protoplanetary discs around stars like Vega
- Protostars
- Ultraluminous infrared galaxies such as Arp 220,
- Dust tori around active galactic nuclei
- Quasars
IRAS was followed by two observatory missions, the Infrared Space Observatory (ISO), launched by ESA in 1995, and the Spitzer Space Telescope, launched by NASA in 2003.
ISO’s 3 to 200 micron spectroscopic capability gave great insight into the nature of interstellar dust and gas in galaxies.
It also allowed astronomers to determine whether infrared radiation in active galaxies was powered by the formation of stars or massive black holes.
ISO demonstrated that interstellar dust has a rich spectrum of emission features between 8 and 20 microns, due to the effects of large molecules called polycyclic aromatic hydrocarbons, which are found on Earth in car exhausts.
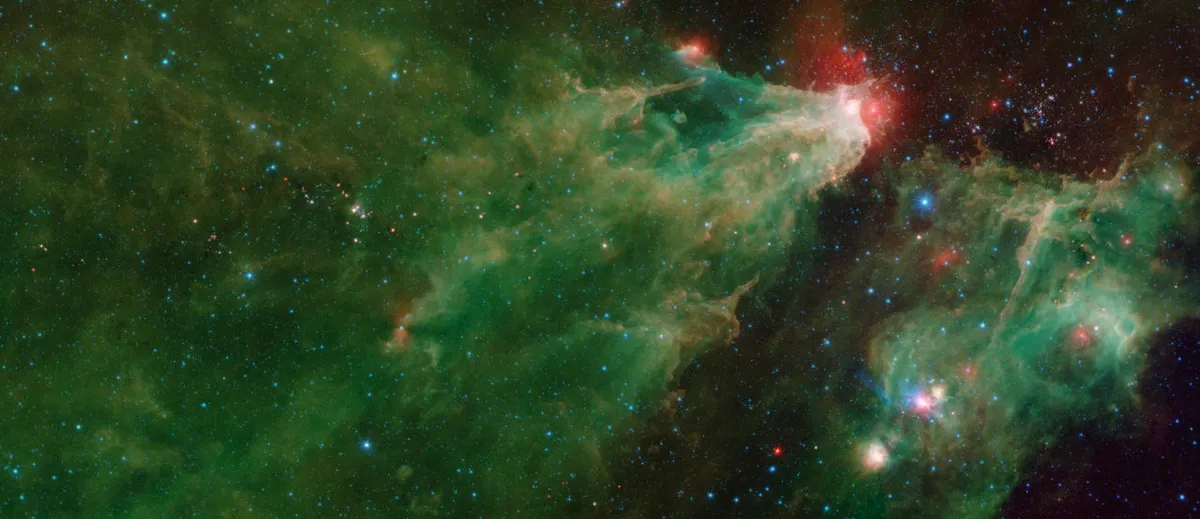
The Spitzer Space Telescope, the fourth and last of NASA’s ‘Great Observatories’ was an 85cm (33-inch) cooled telescope, with three instruments spanning wavelengths from 3 to 180 microns.
The satellite trailed the Earth in a Sun-centred orbit similar to Earth’s, which allowed the telescope to escape Earth’s heat and to cool passively to an ambient temperature of 30 to 40 Kelvin (-240ºC to -230ºC), thereby saving the amount of helium coolant that has to be carried.
In terms of understanding more about the Universe through infrared astronomy, all eyes are now on the James Webb Space Telescope, which is giving astronomers unprecedented views beyond visible light.

Types of infrared
Infrared is subdivided into four sub-bands:
- Near infrared, stretching from 0.8 to 3 microns
- Mid infrared, from 3 to 30 microns
- Far infrared, from 30 to 200 microns
- Sub-millimetre infrared, from 200 to 1,000 microns
In each band we see different sources of radiation.
Comparing infrared with visible light
Let's take a look at how infrared astronomy works by comparing different views of galaxy M81.
Visible light

In this optical view of galaxy M81, we see the young, massive blue stars in the spiral arms, as well as the old red stars of the bulge. This was taken from the ground-based Kitt Peak Observatory.
Near infrared
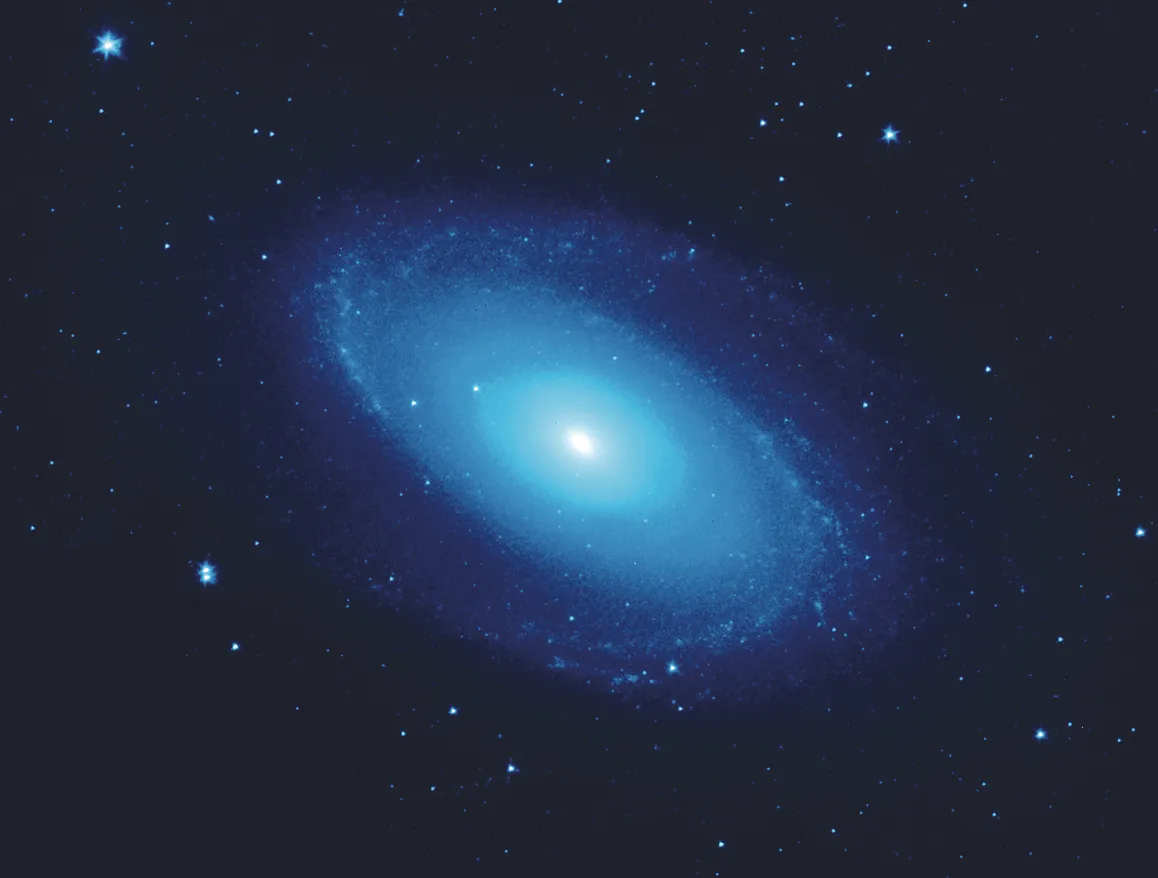
In the near infrared we see light from old stars, like red giants, with surface temperatures around 3,000 Kelvin (2,700ºC).
The near infrared image of M81 is dominated by old stars, so the bulge is very prominent. This Spitzer image is virtually unaffected by the obscuring dust.
Mid infrared

In the mid infrared we see emission from hot dust, with temperatures from 300 to 1,000 Kelvin (30 to 700ºC).
This is also where the objects of the inner Solar System – the inner planets, asteroids and comets – emit their thermal radiation (we also see these at optical wavelengths through their reflected sunlight).
In the mid infrared image of M81 we see the emission from dust clouds, where new stars are forming, so the spiral arms are again prominent. Alongside the optical view, the difference is striking.
Far infrared
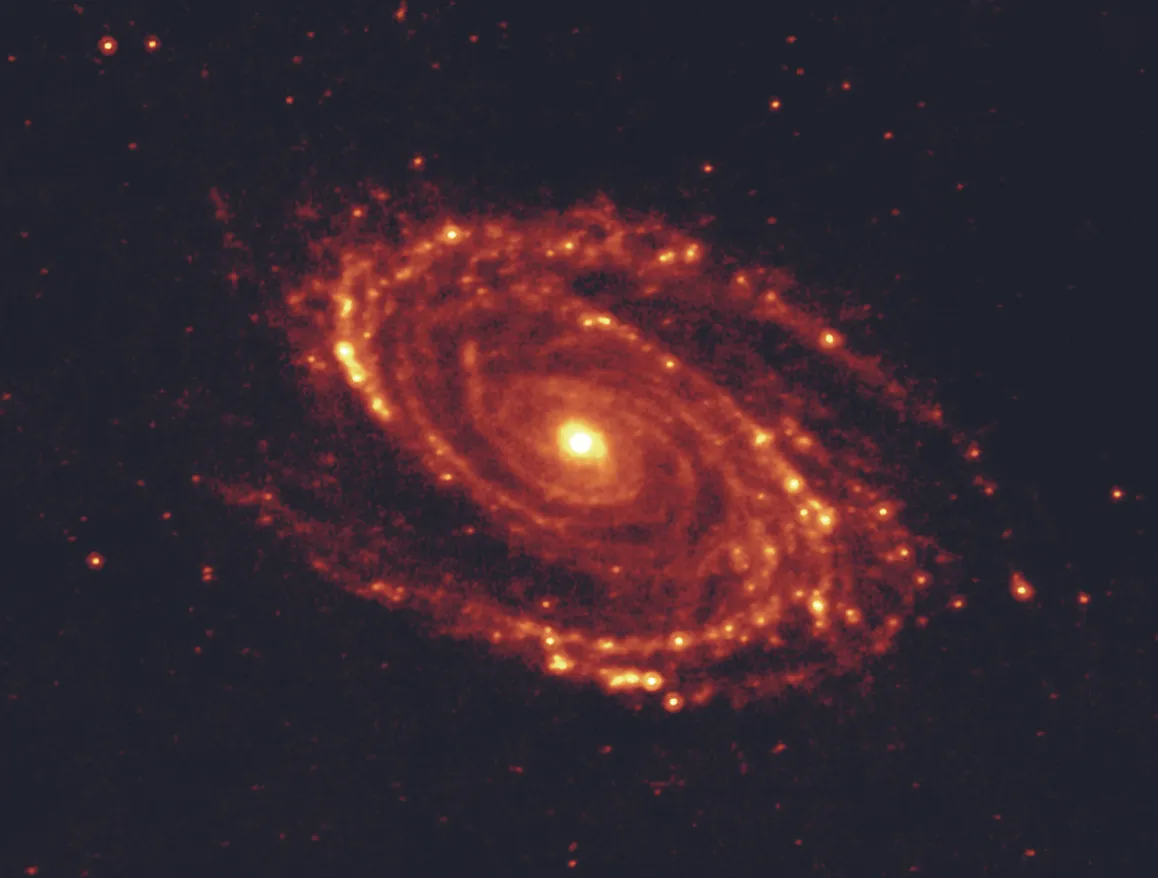
In the far infrared we tend to see cooler dust, at 30–100 Kelvin (-240 to -170ºC). This is where the thermal emission from dusty galaxies tends to peak.
In the far infrared image of M81 we see the cooler dust, both in the star-forming clouds and in the more diffuse interstellar medium. Clumpy knots show where stars are being born.
Submillimetre
In the submillimetre we find that cool objects, with temperatures in the range 3–30 Kelvin (-270 to -240ºC), tend to be prominent. Dusty galaxies at high redshift have the peak of their emission shifted from the far infrared to the submillimetre.
Timeline history of infrared astronomy
1800
William Herschel detects IR radiation by passing sunlight through a prism and measuring each colour’s temperature. It’s highest beyond the visible spectrum.
1856
Charles Piazzi Smyth, Scotland’s Astronomer Royal, detects IR radiation from the Moon while in Tenerife. Better observations are possible at higher altitudes, proving that the atmosphere absorbs infrared.
1948
Infrared observations by Adriaan Wesselink show that the Moon is covered with a fine dust, years ahead of the Apollo missions between 1969 and 1972, which increased our knowledge of the Moon still further.
1950s–1960s
Harold Johnson develops infrared detectors to study the infrared radiation of stars at the McDonald Observatory in Texas. He also devises a system to measure infrared magnitudes.
1969
Gerry Neugebauer and Robert Leighton do the first infrared survey of the sky from Mount Wilson Observatory. They found about 20,000 sources, including star-forming regions, galaxies and stars.
1983
The Infrared Astronomical Satellite (IRAS) becomes the first IR space mission. This US-Dutch-British project is a success and inspires NASA and ESA to approve new space-based observatories.
1993
An infrared telescope is set up in Antarctica. The colder temperatures found there make the South Pole Infrared Explorer (SPIREX) more sensitive to incoming infrared radiation.
1995
Europe launches the Infrared Space Observatory (ISO). It lasts for two-and-a-half years. A highlight is the beautiful IR spectra of stars, galaxies and interstellar dust and gas it captures.
2003
NASA launches the Spitzer Space Telescope, with advanced detectors generating images of galactic nebulae and some of the most distant galaxies.
2005
Light from a planet outside our Solar System is directly detected for the first time. Spitzer captures the infrared light from two planets over 700ºC, both so-called ‘hot Jupiters’.
2006
The Japanese Space Agency (JAXA) launches Akari, first known as IRIS and then ASTRO-F.
Its first images of galactic nebulae demonstrate its vastly superior angular resolution compared to IRAS in the 1980s.
2022
The launch of the James Webb Space Telescope, the latest mission that will give astronomers an unprecedented view of the Universe in infrared.