Astronomy is the science of very large objects: planets, stars, galaxies and the Universe as a whole.
In contrast, particle physics deals with the smallest things we know: the elementary building blocks of matter, particles smaller than an atom.
With such a difference in scale, the two disciplines may seem to have little in common.
However, they turn out to be inextricably linked. Here we’ll explore the basics of what has become known as astroparticle physics.

To study the geology of the Moon, you don’t need to know about the behaviour of individual elementary particles.
But astronomers wouldn’t understand the evolution of stars without knowledge of atomic processes like nuclear fusion.
Supernova explosions would not happen were it not for the weird properties of neutrinos.
Active galactic nuclei, powered by supermassive black holes, spew out the most energetic protons and electrons we have ever observed.
And right after the Big Bang, our Universe was one big particle experiment.
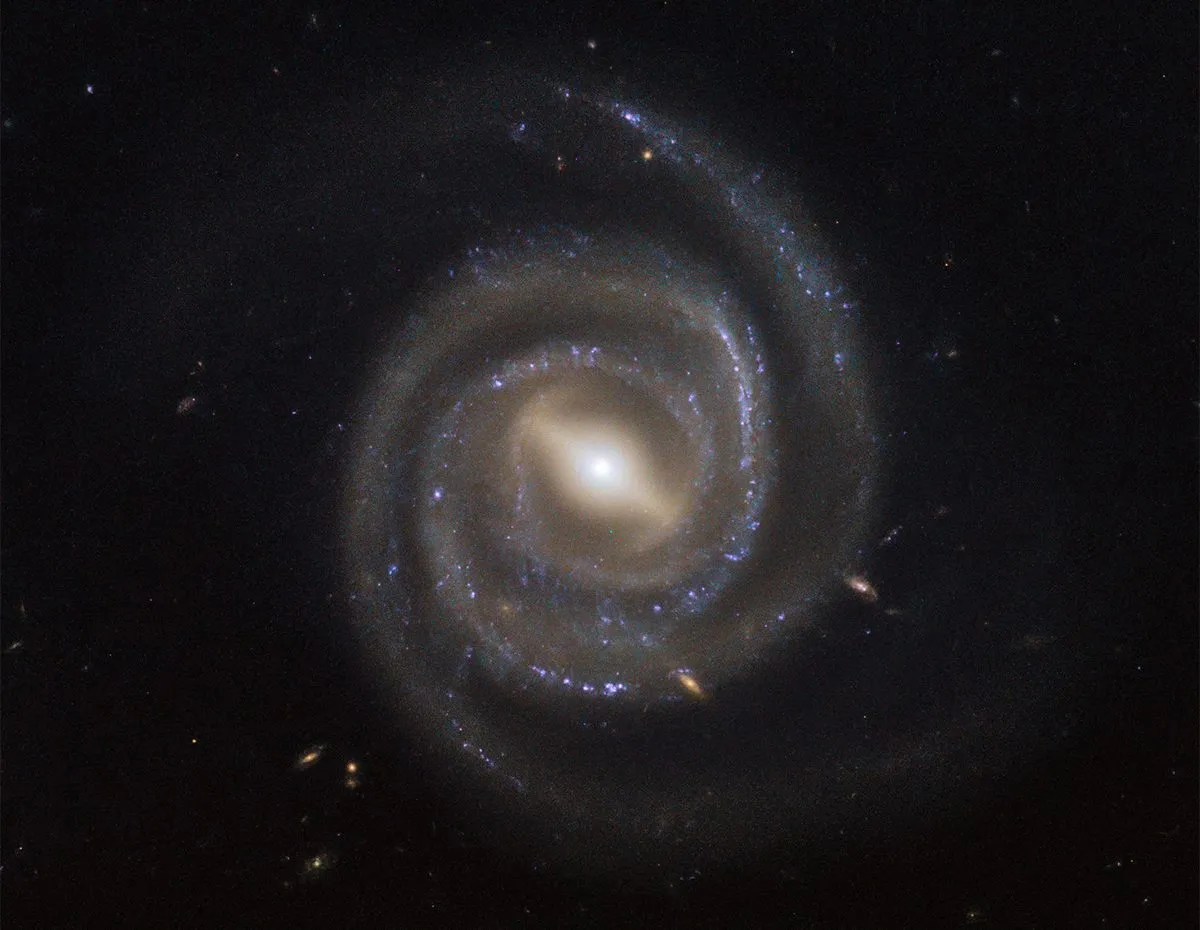
A short history of particle physics
Ancient Greek philosophers assumed that everything in nature consisted of just four basic elements: earth, water, air and fire.
As long ago as the 5th century BC, Democritus of Abdera further suggested that all matter consists of fundamental building blocks, which he named atoms, from the Greek word for ‘indivisible’.
But it wasn’t until the 19th century that we arrived at the modern view of atoms as the smallest representatives of the dozens of chemical elements in the periodic table.
Moreover, physicists discovered that atoms – despite their name – are not actually indivisible. Instead, they consist of even tinier particles.

The Standard Model
Today, we know that the material world is like a huge Lego edifice, built from just three different elementary particles: up quarks, down quarks and electrons.
Since quarks are usually bound up in nuclear particles known as protons (two up quarks and one down quark) and neutrons (two downs and one up), it’s also fair to say that everything in the Universe consists of protons, neutrons and electrons.
Atomic nuclei contain various numbers of protons and neutrons; the number of protons determines what chemical element we’re dealing with.
Surrounding the nucleus is a cloud of electrons.
Electrons, protons and neutrons were only discovered in 1897, 1917 and 1932, respectively.
A fourth elementary particle, the almost massless neutrino (which is not part of the material world but has an independent existence) wasn’t found until 1956.

Over the subsequent decades, particle physicists developed their successful Standard Model, describing not only the building blocks of matter, but also their mutual interactions through four fundamental forces: the strong and weak nuclear forces, the electromagnetic force and gravity.
Surprisingly, it has turned out that the four elementary particles mentioned before are not the final word.
Nature knows of eight additional particles that are very much like the up quark, down quark, electron and neutrino, except they’re more massive.
And there’s another important difference: particles containing one of these four heavyweight quarks (labelled strange, charm, bottom and top) are not stable.
Once they are created in some energetic process (see above for the relation between mass and energy), they immediately fall apart into less massive particles – a process known as decay.
The same is true for the hefty cousins of the electron: the muon and the tau.
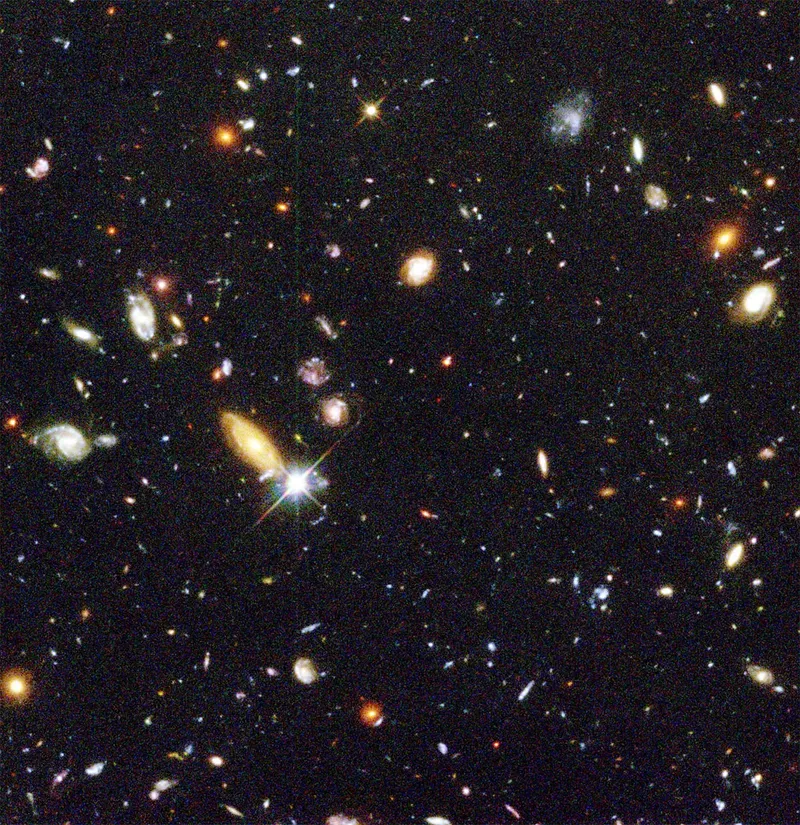
Where astronomy comes in
That’s why particle physicists need the Universe.
While astronomers can’t do without knowledge of the subatomic world to really understand stars, supernovae, active galaxies and the Big Bang, particle physicists use the cosmos as a natural laboratory where ‘experiments’ are played out on a much more energetic scale than any terrestrial particle accelerator could possibly reproduce.
As Astronomer Royal Sir Martin Rees once wrote: "The cosmos provides the only laboratory where sufficiently extreme conditions are ever achieved to test new ideas on particle physics."
Likewise, particle physics experiments here on Earth shed light on the biggest mysteries of the Universe.
Mass and energy
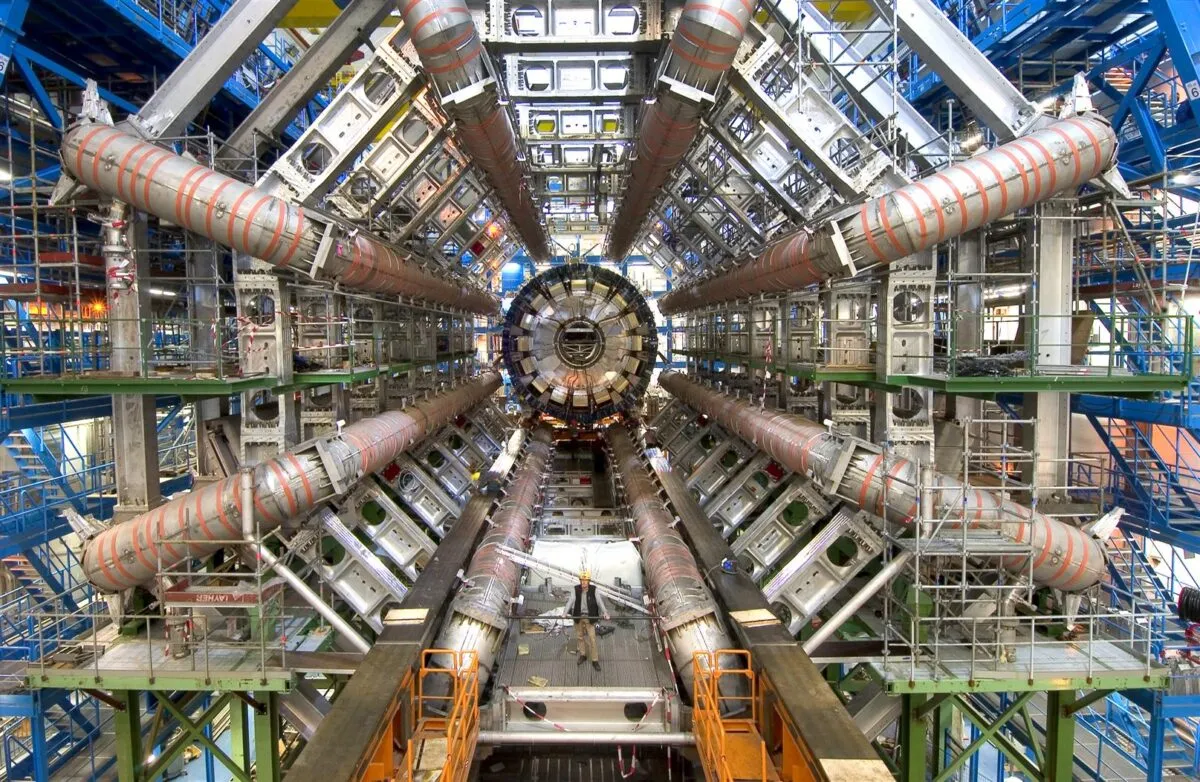
Subatomic particles don’t weigh much.
The mass of a proton (basically the nucleus of a hydrogen atom) is a mere 1.672 x 10-24g – that’s a billionth of a billionth of a gram (1.672 yoctogram).
An electron is another 1,836 times less massive. Instead of using yoctograms, particle physics uses units of energy to denote particle masses.
After all, according to Einstein’s equation E = mc2, mass and energy are inter-changeable.
Particle physicists use the electron volt, the energy gained by one electron as it’s accelerated through one volt.
In these units, the proton has a mass of 938,272,081 electron volts, or about 938 megaelectron volts (MeV). The electron weighs in at 511 kiloelectron volts (keV).
Expressing mass in units of energy has a big advantage.
Through E = mc2 (or m = E/c2), new short-lived particles can be created from the energy of a particle collision in an accelerator such as the Large Hadron Collider (LHC) at CERN in Geneva, Switzerland.
Thus, the available energy tells you the maximum mass of particles that can be produced.
For instance, the LHC was the first machine powerful enough to produce the long-sought Higgs particle (in 2012), which has a mass of just over 125 gigaelectron volts (GeV).
What other aspects of physics and astronomy would you like to see primers on? Let us know by emailing contactus@skyatnightmagazine.com