Black holes might well be nature's most mysterious - and misunderstood - phenomena.
While they are often thought of in science fiction and space movies as portals to another dimension, or huge cosmic vacuum cleaners that suck up anything that strays too close, they are now known to expel powerful jets of cosmic matter into space, and can even radiate heat and light energy that can be observed with powerful telescopes.
Astronomers have even managed to capture the first photograph of a black hole, in galaxy M87, revealing even more of the secrets surrounding these colossal cosmic objects.
Questions about black holes abound, and while the answers aren't always definitive, cosmologists have managed to piece together an incredible amount of knowledge, which, considering black holes were largely hypothetical until relatively recently, is a feat in itself.
Below are some of the most commonly-asked questions about black holes and their role in the workings of the Universe.
11 questions about black holes
1
Do all stars become black holes?
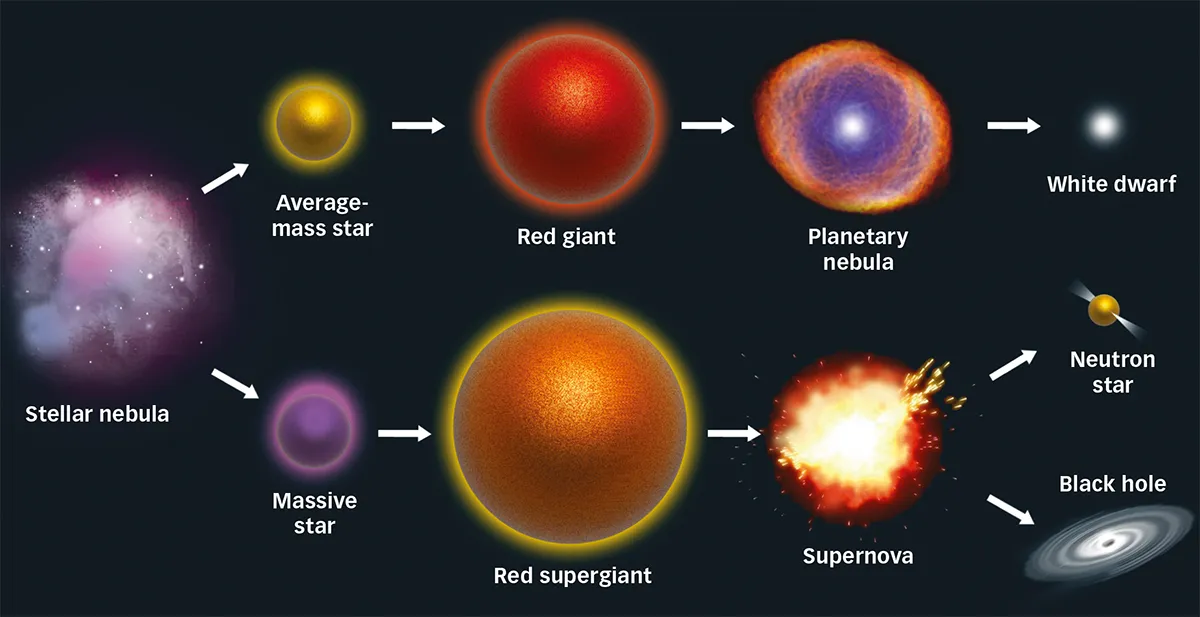
Medium-mass stars, which explode in a supernova and form neutron stars, are thought to be between about eight and 30 solar masses.
Stars less massive than this end their lives relatively gently, leaving behind planetary nebulae and white dwarf stars.
Stars with masses greater than 50 times the mass of the Sun will end up as black holes.
What happens to the more massive stars that fall in the 20-50 solar-mass range is still uncertain, and either a neutron star or black hole could result.
The proportion of the star’s chemical make-up that isn’t hydrogen, or the star’s metallicity, seems to play a key role in its death.
The ultimate fate of medium-mass stars at the high end of the range (20-30 solar masses) depends on the final stages that lead up to the supernova.
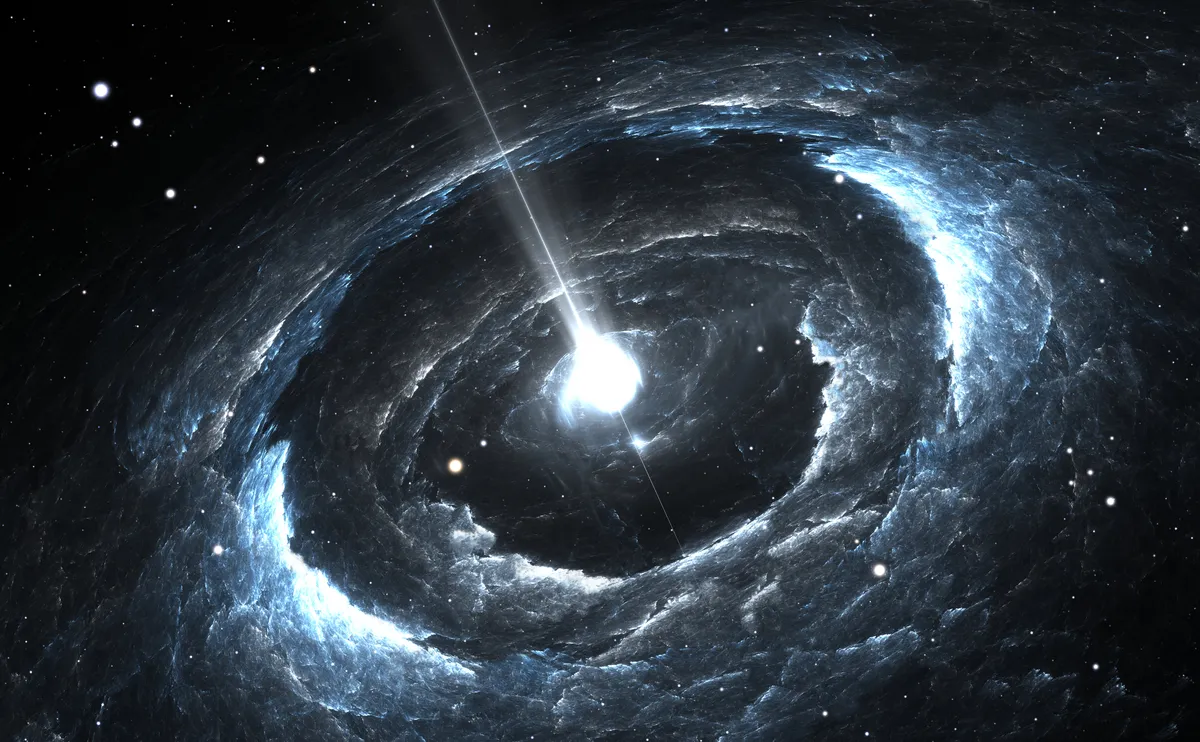
A star loses mass in a variety of ways, primarily via stellar wind, and if enough material is shed, it may be able to lower itself below the threshold and end up as a neutron star.
In 20-30 solar mass stars, stellar wind mass loss can be very high – a few hundredths of a solar mass a year in extreme cases.
So the metallicity of the solar wind, velocity and density are key factors in determining whether a star will shed enough weight to avoid ending up as a black hole.
The supernova explosion ejects huge quantities of material (several solar masses worth), crushing the core down to incredible densities, creating a neutron star.
This star is supported by the pressure of the neutrons within it. However, above a certain mass of about twice that of the Sun, but certainly no more than three times, this pressure can’t hold off the force of gravity, and the neutron star collapses in on itself to form a black hole.
2
Are black holes flat?

Not really. A black hole forms when a massive body, such as a big star at the end of its life, shrinks under its own gravity down to a point-like ‘singularity’.
Since all that remains is a region of grossly warped space-time, pretty much like a bottomless well, defining the shape of a black hole is not straightforward.
Physicists visualise an imaginary membrane, or ‘horizon’, which surrounds the singularity.The horizon is the point of no return for in-falling matter.
And light originating on the horizon can never climb out of the gravitational well of the hole, which is why a black hole is black.
This horizon is spherical, like a perfect soap bubble, and certainly in the case of a non-rotating, or Schwarzschild, black hole, we can say the hole is shaped like a sphere.
However, in the case of a rotating, or Kerr, black hole, there is another surface outside the horizon.
Between this ‘static limit’ and the horizon, space swirls round so fast that even light must rotate with the hole.
The static limit bulges at the equator like a spinning body, so a rotating hole can be said to have the shape of an oblate spheroid (a ‘squashed’ sphere).
3
Can we see both sides of a black hole?

Even if we could see black holes directly, they are not holes in space in the sense that you could see through them like you can see through a polo mint.
They are regions of grossly warped space-time and they blot out stuff behind them like a solid, opaque object.
On the other hand, their gravity can bend light like a lens, creating multiple images of a background object – a quasar, for example. This is known as gravitational lensing.
So, in a sense you can see a distorted image of something that is far beyond.
4
What's the temperature of a black hole?
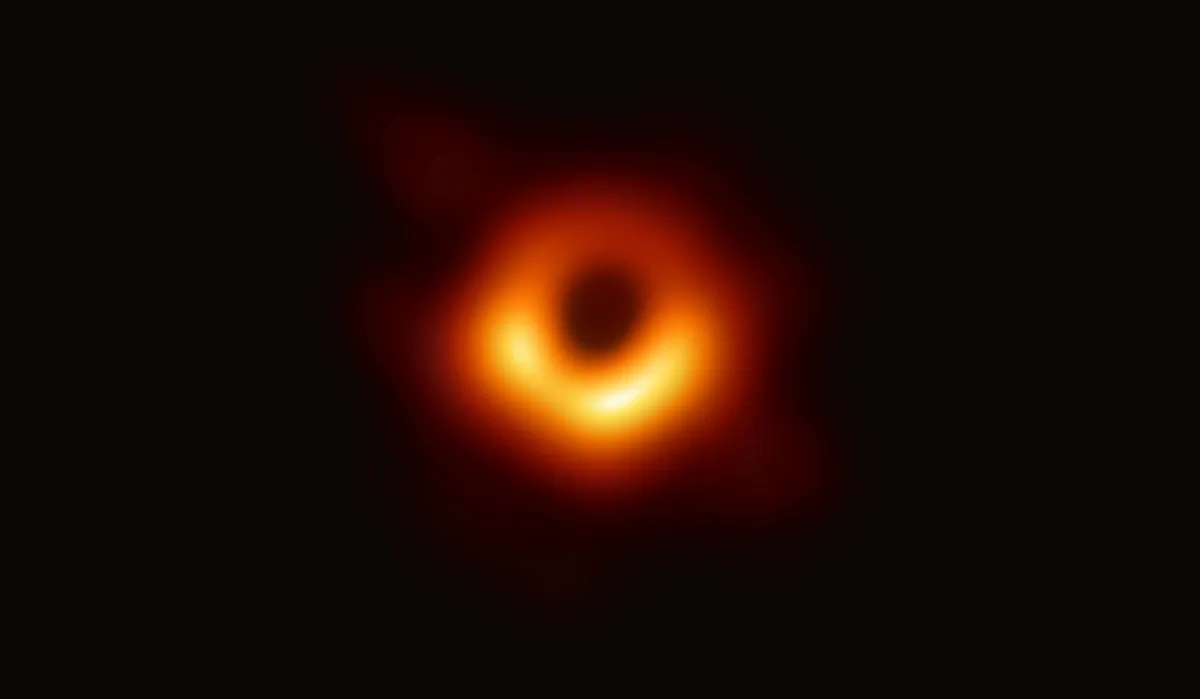
Strictly speaking, this question has no meaningful answer. The event horizon – an imaginary surface cloaking a black hole – marks the point of no-return for in-falling light and matter.
Consequently, nothing – not even heat – can escape it.
But, if no heat can reach the outside Universe from inside the horizon, the interior can have no meaningful temperature for the outside Universe.
Nevertheless, a black hole does have a temperature, something which took physicists by surprise.
The first hint was found by Israeli physicist Jacob Bekenstein, who speculated in 1972 that the surface area of the event horizon represents the ‘entropy’ of the black hole.
Entropy is a quantity intimately connected with thermodynamics, so everyone was mystified.

All became clear when Stephen Hawking discovered that microscopic ‘quantum processes’ cause a black hole to radiate a continual hail of subatomic particles.
These do not come from inside the black hole – that would be impossible. Incredibly, they appear to be conjured out of the apparent vacuum that surrounds the event horizon.
Because of this ‘Hawking radiation’, a black hole is not completely black. Like anything else that radiates, it has a temperature.
For a stellar-mass black hole, that temperature is mind-bogglingly small, though for a microscopic black hole it would be huge enough to cause it to radiate so much heat that the hole would quickly disappear.
5
How does Hawking radiation escape black holes?
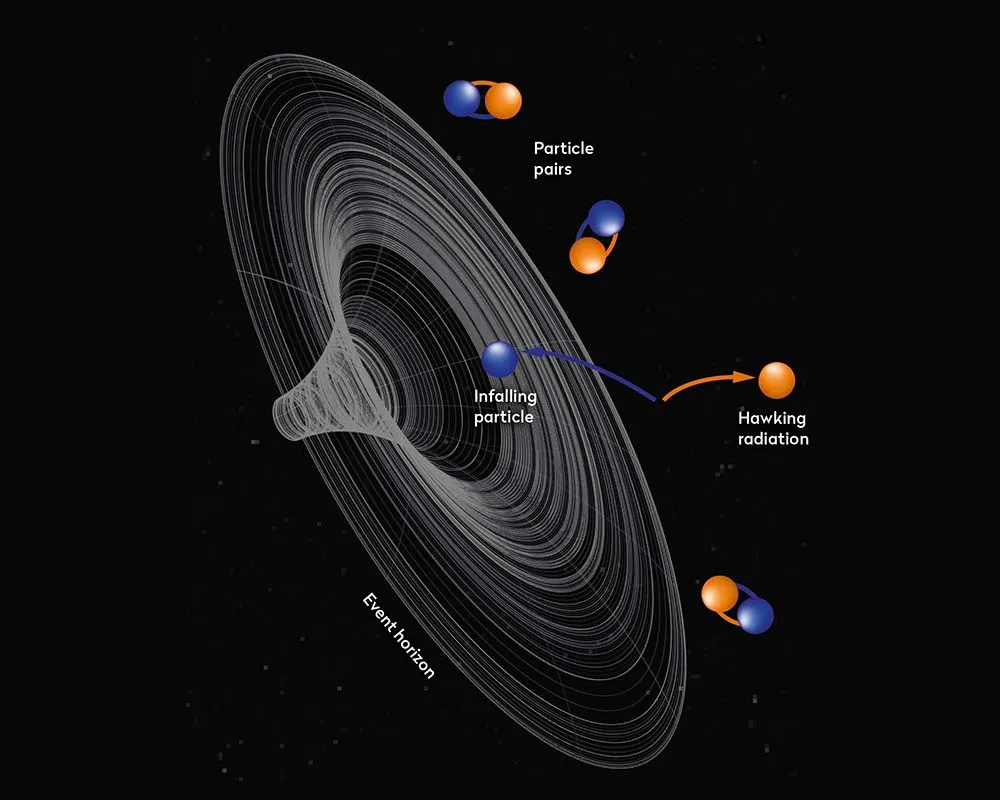
Hawking radiation does not escape from a black hole. That would imply that it originated inside a black hole and somehow broke free.
However, the defining characteristic of a black hole is that nothing – not even light – can escape its gravitational clutches.
Hawking radiation does not escape from a black hole because it wasn’t inside in the first place.
Instead, it is created in the region just outside the ‘event horizon’, the point of no return for in-falling matter.
This is how it works: according to quantum theory, the vacuum is not empty but is a seething cauldron of energy.
Subatomic particles are constantly popping into existence along with their anti-particles – for example, electron-positron pairs.
Nature turns a blind eye to this creation of matter out of nothing as long as the particles and antiparticles disappear, or ‘annihilate’ each other within a split second.
All is well, except near the event horizon of a black hole.
Here, it is possible for one member of a particle-antiparticle pair to fall into the hole.
The remaining particle is left without a partner to annihilate itself with.
It is therefore elevated from a particle with a fleeting existence, known as a ‘virtual’ particle, to a real particle that has a permanent existence.
Subsequently, it flies away from the hole in the form of Hawking radiation.
6
Could jets from our galaxy's black hole cause an extinction event?
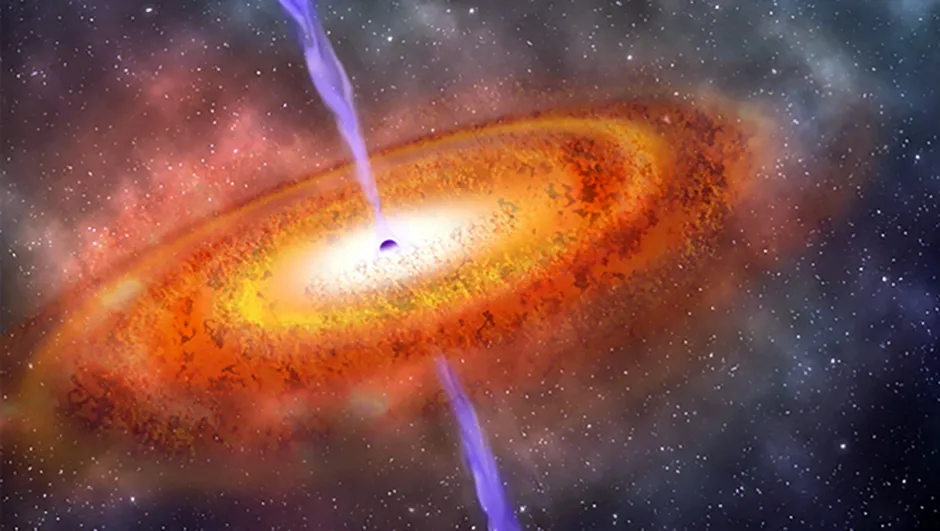
The heart of our Galaxy does indeed harbour a supermassive black hole.Known as Sagittarius A, it weighs about 2.6 million times the mass of the Sun.
Although this sounds impressive, it is more than 1,000 times less massive than the supermassive black holes that drive the most powerful quasars (the bright hearts of distant galaxies).
Some quasars display spectacular, thread-thin ‘jets’ of ultra high-speed subatomic particles that stab out from the poles of their central supermassive black holes.
However, Sagittarius A is currently inactive and has no such jets. If it did have them in the past, they would have been thousands of times weaker than those produced by a powerful quasar.
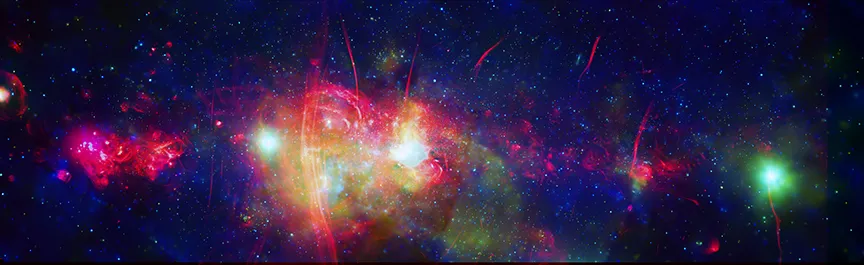
In fact, the jets would have been so weak that, at the 26,000 lightyear distance of the Earth, they would have been unable to damage the Earth’s ozone layer, which would create a lot of problems for life on Earth.
That would require a supernova going off within about 30 lightyears of Earth.
On the other hand, there may never have been or never will be such a threat, because the spin axis of Sagittarius A and the Milky Way are likely to be aligned.
This means that any jets would emerge perpendicular to the disc of the Galaxy in which all stars orbit, including the Sun.
Jets would only come our way if the spin of Sagittarius A had been changed in the past, perhaps by an encounter with another supermassive black hole.
7
Do black holes collide?
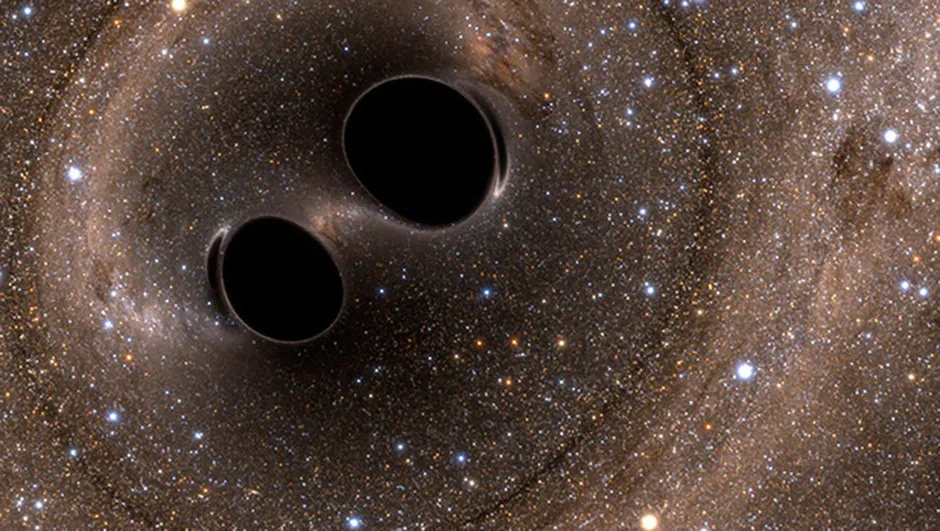
As with much black hole research, evidence for collisions comes from data obtained over a variety of wavelengths, but primarily from X-ray observations.
The gravitational forces in black hole collisions can accelerate material to huge velocities.
When this material is compressed it reaches temperatures of millions of degrees, emitting most of its electromagnetic radiation in the ultraviolet, X-ray and even gamma-ray regions.
Black holes are thought to exist in a variety of sizes, ranging from stellar mass (a few times the mass of the Sun) to supermassive (millions or billions of solar masses), and astronomers think their observations provide evidence for collisions of both extremes.
The collision of stellar-mass black holes is one production mechanism for gamma-ray bursts, enormous blasts of high-energy radiation, lasting typically no more than a few seconds.
At the other extreme are colliding galaxies. It’s thought most (or even all) galaxies contain supermassive black holes at their cores.
As galaxies collide, these giants may orbit each other and eventually merge.
The merging galaxy system NGC 6240 appears to contain two nuclei, clearly visible in radio, infrared and optical images, and X-ray observations suggest these are twin black holes about 3,000 lightyears apart.
Astronomers using very long baseline interferometry have studied the elliptical galaxy 0402+379 and found it contains two supermassive black holes (totalling over 150 million solar masses) only 24 lightyears apart – more than 100 times closer than the closest pair previously observed.
Gravitational wave detectors should soon start to pick up the distortions in space-time created by the merging of black holes.
8
Can light go into orbit around a black hole?
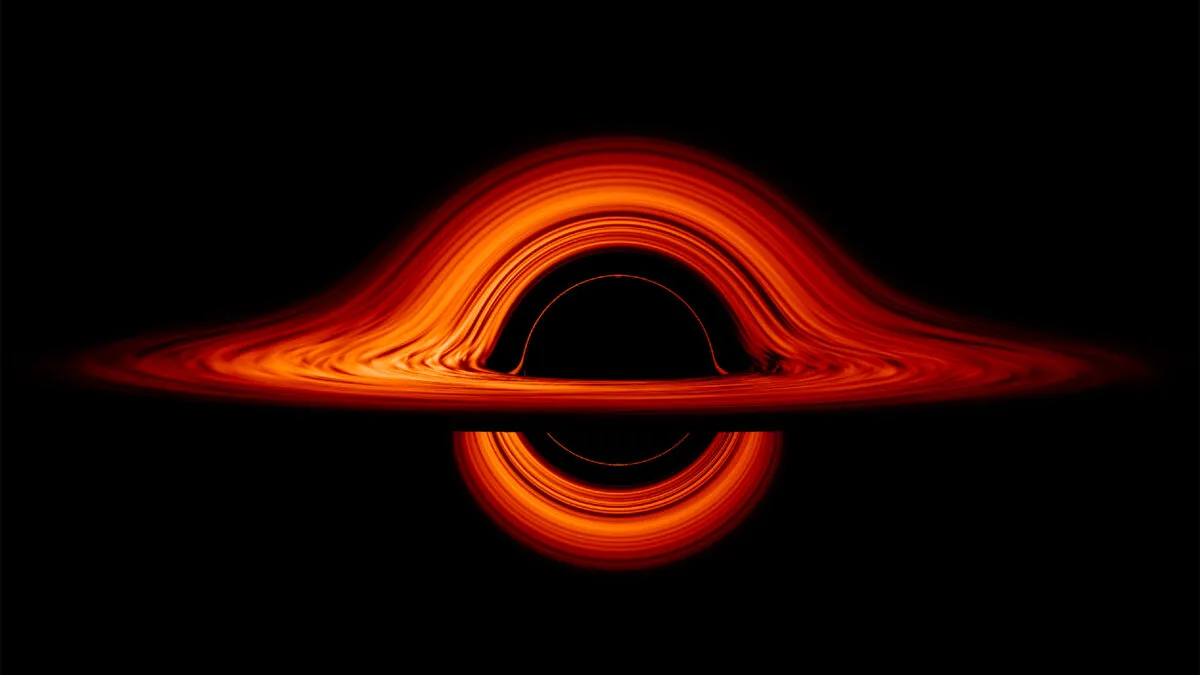
As Isaac Newton realised, the natural tendency of a body in space is to travel at a constant speed in a constant direction forever.
However, if such a body passes near a much bigger mass, its trajectory is bent towards it by the bigger mass’s gravity.
If the gravity of the larger mass is strong enough, or if the velocity of the small body is small enough, its trajectory can be bent into an ellipse or circle around the larger body. In short, it can become trapped in orbit.
Albert Einstein discovered that particles of light, which are called photons, have an effective mass.
However, they are travelling so fast – 300,000km/s – that no star has a strong enough gravitational pull to trap them in orbit.
Only the strongest source of gravity known, a black hole, can do that. At a distance of 1.5 times the ‘Schwarzschild radius’ – the black hole’s effective radius – photons can circle endlessly.
So light can orbit around a black hole. Consequently, if you could hover there in a spaceship and look in a direction perpendicular to the black hole you’d see the back of your own head!
9
What's at at the centre of a black hole?
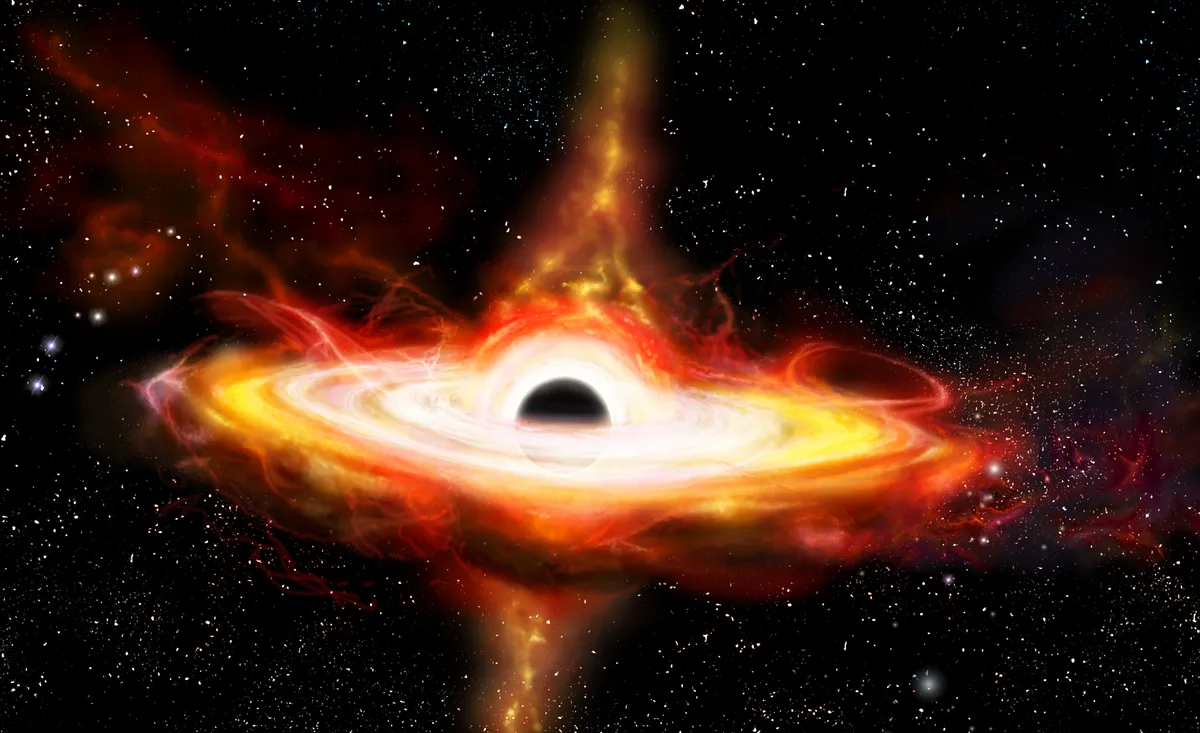
When a massive star shrinks catastrophically under its own gravity to form a black hole, Einstein’s theory of gravity predicts that the matter of the star is squeezed down to a point of zero volume and infinite density known as a ‘singularity’.
In other words, the matter vanishes and is crushed out of existence.
Some speculate that it reappears in another Universe, fountaining out of empty space as a ‘white hole’.
The black hole, empty of matter, is therefore made of nothing other than space and time. Imagine it as a bottomless well in the fabric of space-time.
A singularity, however, is a monstrous thing that indicates that a theory has been stretched beyond the point where it has anything sensible to say.
As yet, a better theory – a so-called quantum theory of gravity – is not within our grasp.
Nevertheless, it is expected to show that something happens before gravity becomes infinite, some softening of the singularity to leave some kind of quantum relic.
This is expected to be an extreme and violent object.
Although its properties will not be infinite, they will be closer to infinity than anything else in the Universe.
So at the heart of a black hole there may well be a speck of ultra-dense matter, or at least a speck of ultra-dense something.
10
Do black holes consume each other?
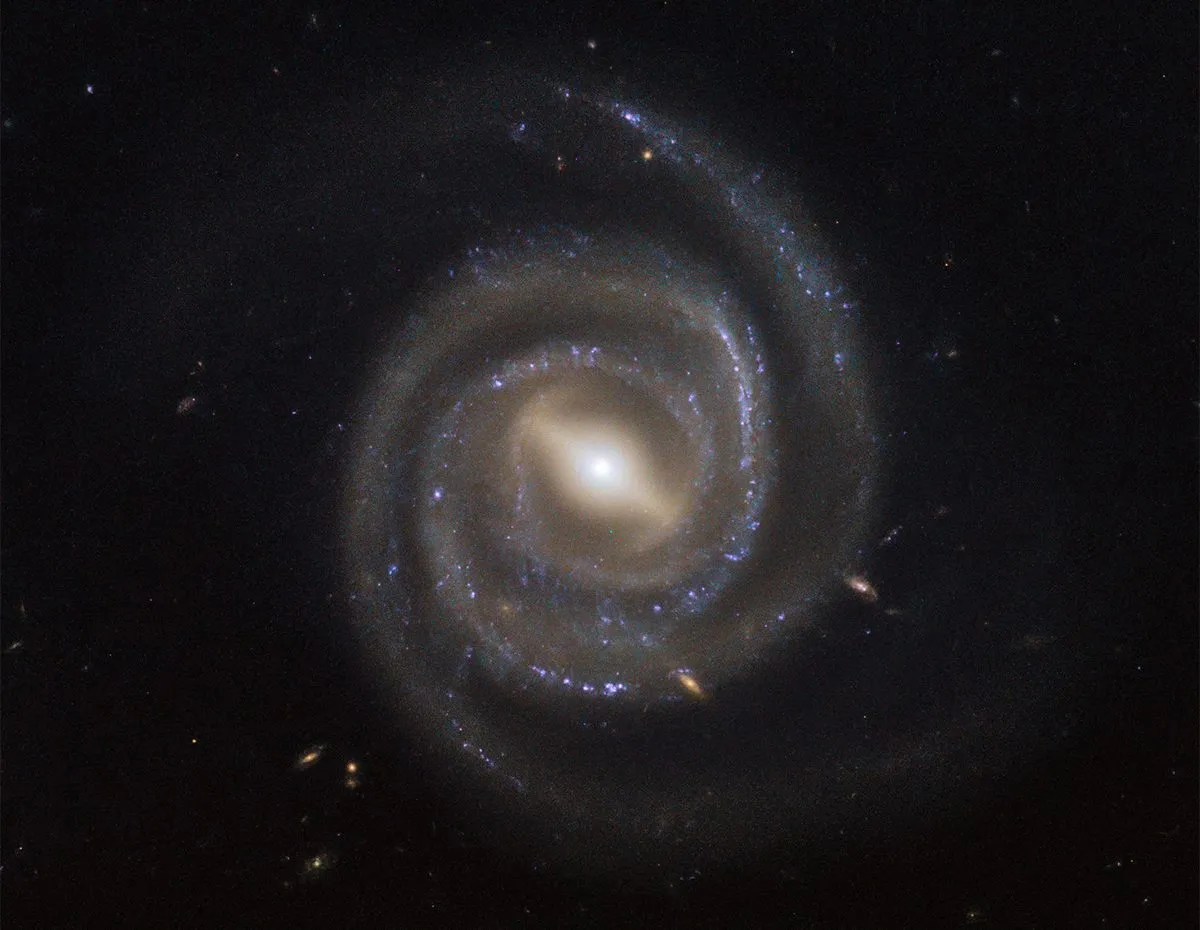
Not only are black holes believed to eat each other, but such cannibalism is thought to have played a key role in the build-up of ‘supermassive’ black holes that appear to lurk in the heart of every galaxy.
Such black holes range in size from tiddlers like the 2.6 million solar-mass hole at the centre of our Milky Way, up to ones pushing 10 billion solar masses.
Nobody knows precisely how they form, but a good guess is that they were created by the merger of stellar-mass black holes in the kind of super-dense star clusters thought to exist in galactic cores.
Thereafter, galaxies collide and their central supermassive black holes join up. This process is thought to have built up galaxies like our Milky Way.