The Square Kilometre Array (SKA) is set to be the next stage in the field of radio astronomy which, rather than observing optical light, focusses on the radio waves emitted by objects in space like stars and galaxies.This technique enables astronomers to see through dust and gas that might otherwise obstruct observations, but also to see objects and processes that are invisible to the human eye.
The Square Kilometre Array will consist of thousands of dishes spread across Western Australia and southern Africa, and construction is due to begin over the coming years.
Due to SKA’s incredible seeing power, it should be able to observe light from the distant Universe, effectively looking back in time to shortly after the Big Bang.
Even though the dishes may be separated physically, the technology is able to combine their data-collecting power to create one huge radio telescope observing one square kilometre of the sky.
Deep in the heart of rural Cheshire in the north of England, the world famous Jodrell Bank Centre is the science headquarters of this global project , which will see two enormous telescopes built in South African and Australia.
It is hoped the Square Kilometre Array will uncover many of the secrets of the Universe, from the birth of the first stars and galaxies to cosmic unknowns like dark matter and dark energy, or the nature of gravitational waves.

We spoke to Professor Philip Diamond, the General Director of SKA based at the Jodrell Bank headquarters, to find out more about this ambitious project.
How did the SKA project come about?
The very first thoughts that resulted in the SKA concept occurred in the late 1980s and early ‘90s.
Radio astronomers were thinking about the next big science questions and one thing that is uniquely accessible to radio astronomy that is not accessible to other types of astronomy, is the so-called hydrogen line.
Hydrogen is the most common element in the Universe, and is the basic constituent of everything else in the Universe.
Very conveniently for radio astronomers, it has an emission line at a wavelength of 21 centimetres.
As you look further out into the Universe, that wavelength gets longer and longer due to the expansion of the Universe.
To detect hydrogen all the way back to close to the Big Bang we need a massive telescope, so we started thinking about what technologies we would need to be able to deliver it.
And of course, if you build a massive telescope, then all sorts of other science becomes possible.
Over the next 15 years or so these ideas grew organically, and then in the early 2000s various groups around the world began to get funding to develop the technology that was needed: broadband systems, new types of telescope etc.
That was the start of the technology development to support the science ideas.
The ideas continued to develop and just a couple of years ago we published the current science paper for the SKA, which is 2,000 pages long. It’s in two volumes that weigh more than 10 kilograms!
It’s a substantial piece of work put together by 1,200 astronomers from around the world.
That drives the technical design of SKA, which really gelled in about 2013/2014 after a lot of technology development and prototyping systems over the years.
We’re now in the final stages of the detailed design of the SKA.
We’re going to build two telescopes: one that consists of 197 dishes in the Karoo in South Africa, and a second, which will consist of what we call low frequency log-periodic antennas.
These are basically very sophisticated cousins of those old TV aerials we used to have in our houses.
We will have 131,072 of these sitting in the desert of Western Australia, and they will study very low frequency radio waves.
SKA is able to use the distance between two separated radio dishes to effectively create one massive data-collecting area. Is this a new method of astronomy?
The technique is called interferometry, and has been used in radio astronomy since the 1950s and ‘60s.
In fact the late Sir Martin Ryle won the Nobel Prize in physics for defining the technique that we call Earth-rotation synthesis, which uses this technique of separated antennas and relies upon the rotation of Earth to synthesise a much larger dish that is effectively the diameter of the two antennas that are furthest apart.
This is a well-known technique, and you can see a great example of this in the Very Large Array, which is based in New Mexico in the US, and there’s the Atacama Large Millimeter/submillimeter Array, ALMA, in the Chilean Andes, which operates at a very high frequency.
And there’s even one in the UK, which is called eMERLIN and is based here at Jodrell Bank, and that’s got dishes spread across central England.
It’s a well-known technique.
The capabilities of modern fibre optic systems, modern receivers, modern electronics are far superior to what they were back in the 1960s.
We use radio telescopes on different continents and link them via fibre optics back to a central digital processor.
There is even a Russian radio telescope in space, and previously there has been a Japanese radio telescope in space.
These beam their data back down to a ground station.
The first phase of the SKA in South Africa will be nearly 200 dishes in Karoo, with a maximum separation of 150 kilometres, but our ultimate plan is 2,500 dishes spread across 3,500 kilometres all over southern Africa.
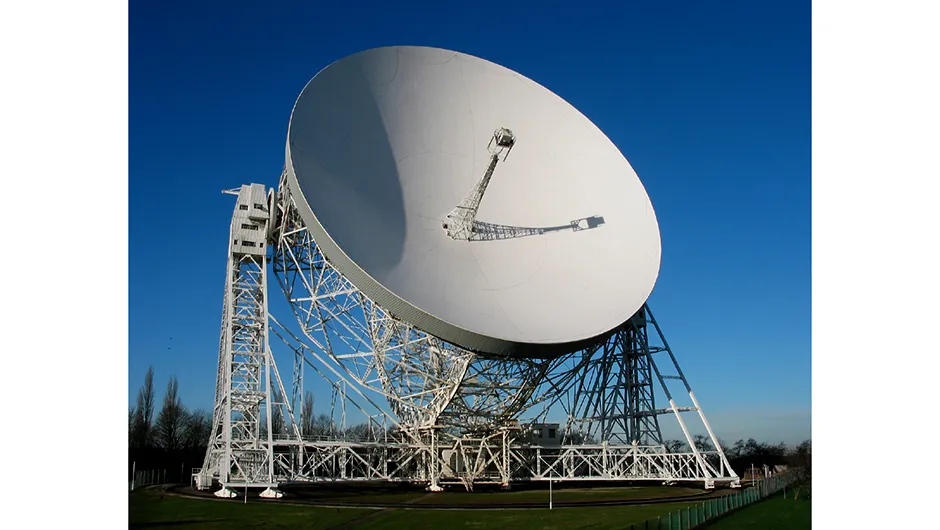
It’s a huge engineering feat, considering the magnitude of the energy and resources that will go into it.
That’s why it’s taken so long to develop the design!
Five years ago we would have struggled, because the data communications technology was not really at the stage where we could use it, but now it is.
The technology behind broadband receivers develops all the time.
But putting in place the infrastructure for SKA: the foundations, the roads, the power, the buildings we will need, the fibre connections, the antennas, this is a big task.
The receiver systems that are on the dish array in South Africa will be cryogenically cooled so we will need liquid nitrogen and we need to be able to transport this and handle it.
We also need to get power to these remote places.
In Australia our colleagues have put in place a two megawatt solar power station with a battery storage system, so it charges and we use that power when we observe at night.
There are so many innovations that have made the SKA possible, but yes it’s a huge engineering challenge.
We’ve had a five-year detailed design programme, we’ve had about 600 engineers around the world in 12-15 different countries working on this design effort, and we’re very close to the end now.
There have also been pathfinding missions to test the water ahead of SKA’s completion.
Yes, there have been. In Australia there are two.
There’s the Australian SKA Pathfinder, which is an array of 36 12-metre dishes, and adjacent to that is what’s called the Murchison Wide Field Array, which is looking at low frequency radio waves.
These don’t look like telescopes at all; they look like 18 inch-high metal spiders actually! But they are radio telescopes.
In South Africa our colleagues have just completed the 64-dish MeerKAT array, which is spread across about 8 kilometres.
This will be fully incorporated into SKA when we add another 133 dishes down there.
What sort of science will SKA be carrying out, and what sort of discoveries might it make?
There are some key things that we will be looking for.
One is a very special type of object in space called a pulsar.
These are the remnants of massive stars that have gone supernova, meaning they have exploded.
What’s left behind is a core of dense exotic matter about 10-15km in diameter that is rapidly rotating as a result of the explosion.
Pulsars have radio jets emanating from them, so they are almost like a lighthouse in the sky.
As the pulsar rotates it beams across the sky and many of these beams happen to be pointed at Earth.
We can detect these pulsars, and what’s fantastic about them is they are superb natural clocks because their rotation is so well-defined.
Nature has essentially provided us with a network of clocks across the sky, and we can use pulsars to measure gravitational waves.
If two supermassive black holes collide somewhere in the Universe, this creates a gravitational wave, and we can see the effects of that wave on this network of clocks.
We also know it’s possible with SKA to peer back to the time just after the Big Bang, and we want to see the first stars switch on, the first galaxies form, and then watch how the structure and the matter of the Universe evolved from the Big Bang until now.
It’s really like a time machine pointing back 13.8 billion years.
We’ll get the best handle on the magnetic Universe, the effects of magnetic fields from all sorts of phenomena: stars, galaxies, the intergalactic medium.
We’ll be doing surveys of gas and star formation, we’ll watch new planets being formed in our own Galaxy.
ALMA in Chile, for example, can observe the dust out of which new planets form, but we’ll be observing the boulders that are the next stage in the formation of planetary systems.
We possibly also have the best chance of detecting any signals from intelligent civilisations if they are out there.
The other big mysteries of the Universe include dark matter and dark energy.
But if we can’t detect them, how will SKA attempt to learn more about them?
The first indications that dark matter existed came from radio astronomy.
If you look at a galaxy in optical light, you can see the stars and the lovely spiral structures, and the shapes of elliptical galaxies too.
The stars in a galaxy provide a fairly well-defined structure, but if you look at the galaxy with a radio telescope, you see the gas, not the stars.
You see that the hydrogen extends much further out than the stars, but when you study the dynamics in detail and look at the motion of the stars, you can weigh the galaxy.
When you do the same with the hydrogen, you find that the two values are very different.
So there must be some matter that we can’t see that’s causing this very dramatic difference.
That was the first clue that dark matter existed.
The huge sensitivity of the SKA, the ability to study hundreds of millions and ultimately a billion galaxies, and their hydrogen; this will give us the tools and the mass of data that we need to be able to help understand the nature of dark matter.
There will also be various ways we can probe the nature of dark energy.
There is a European Space Agency satellite called Euclid that is due to be launched in a couple of years.
This will approach dark energy from the optical infrared wavelength, and we will do similar experiments in the radio.
Putting these results together will put more restraints on the nature of dark energy and together hopefully we can figure out what it is.
When can we hope to start getting the first science results and discoveries?
Our precursors are either operating or are in the final commissioning stages before operations, so they are already delivering science and will become much more productive over the coming years.
We are in the final stage of the detailed design.
I hope we will begin construction early 2020, and we will have a six- or seven-year construction period.
There is a mass of metal and electronics needed and a huge software project that we are putting together.
I would expect the first initial science results to begin emerging in about 2025/2026.
I’ve been working on this project for quite some time, and it’s great to see the very bright light at the end of the tunnel.
Keep up to date with the SKA project at the official website and follow them on Facebook or Twitter.