Why is the Sun important? It is, after all, a nondescript star, differing little from billions in the Milky Way galaxy. It is a star of typical mass, typical colour and luminosity, a middle-aged star.
It is of spectral type G in the range O, B, A, F, G, K, and M that is a sequence of decreasing mass and temperature.
Yet the Sun is special and of interest, simply because of our proximity to it.
From Earth’s orbit, the Sun is over 270,000 times closer than α Centauri A, the nearest night-time star that is otherwise Sun-like.
Our closeness allows us to observe, measure and document its behaviour in detail.
Some basic properties of the Sun
- Mass 2x1030kg
- Radius 700,000 km ≡ 1 AU
- Distance 150,000,000 km
- Luminosity 4x1026W
- Irradiance at Earth 1.365 kW m-2
- Average rotation period 27 days
- Stellar spectral type G2 V
The table above lists some physical properties of the Sun as well as its classification among the stars.
The notation “G2” in the spectral type refers to the Sun’s colour and approximate temperature in the stellar O through M sequence, running for G-type stars from G0 to G8.
The “V” denotes the size of the star, or its “luminosity” class among the stars. It classifies the Sun as a normal dwarf star, currently on the “main sequence” .
The irradiance at Earth is the amount of radiative power received across 1m2 at Earth's orbit, it is over 1kW per metre2.
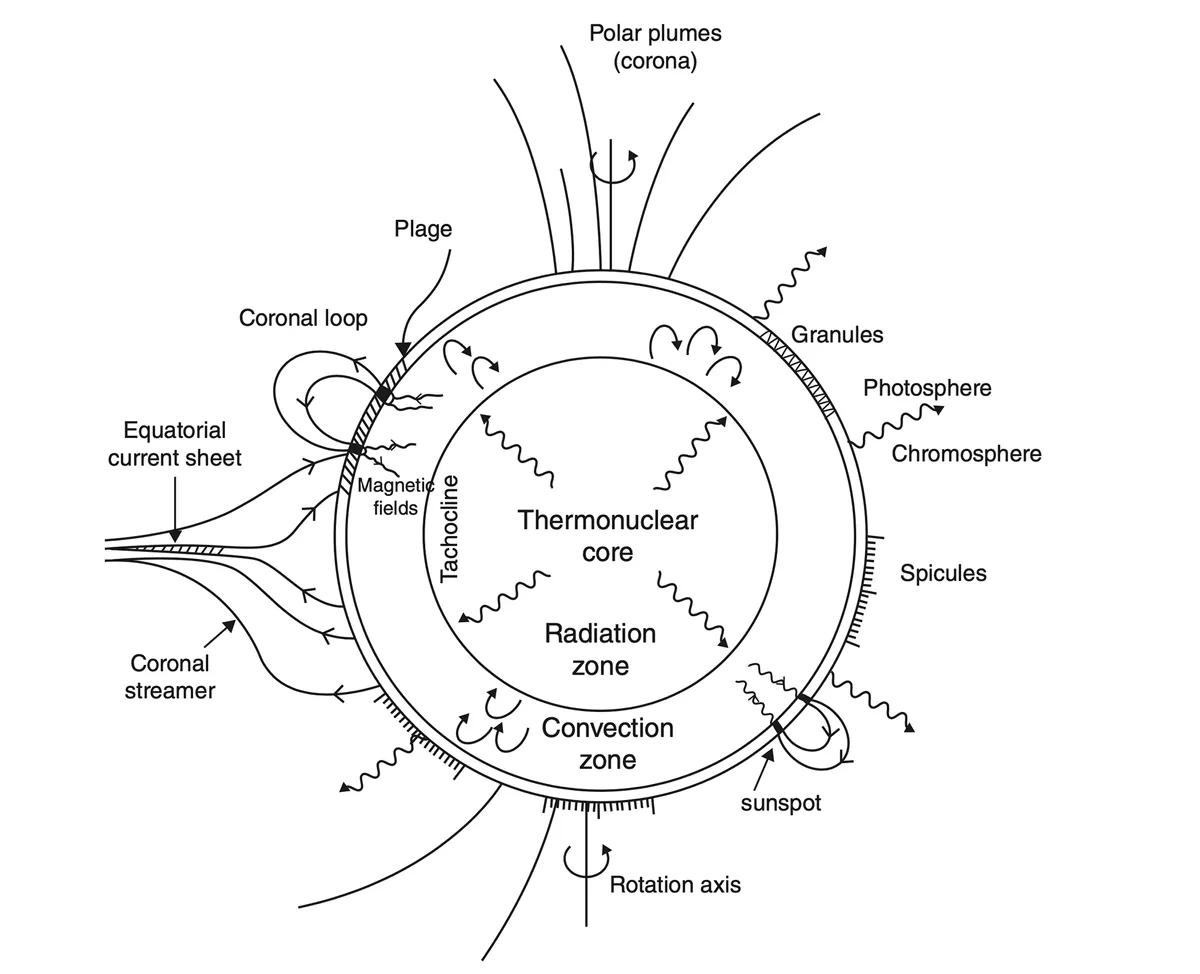
The Sun’s proximity also makes it important as a laboratory for studying basic physical phenomena that can occur under conditions totally unreachable in our laboratories.
Solar studies inform us of nature operating on the enormous scales encountered across the Universe.
It exhibits remarkable phenomena that we take for granted, such as sunspots, the corona, flares, the solar wind, and coronal mass ejections (CMEs).
Similar phenomena occur in different guises in many astrophysical plasmas in which plasma motions and magnetic fields interact.
Yet we could not anticipate any of these phenomena from first principles. The Sun is thus a proving ground where we can test theories of “magnetised plasmas” of importance also in our quest to find safe power from nuclear fusion.
Using the Sun as our laboratory, we can try new measurement techniques in astronomy, and pit them against theories and models, in our quest to understand the entire Universe.
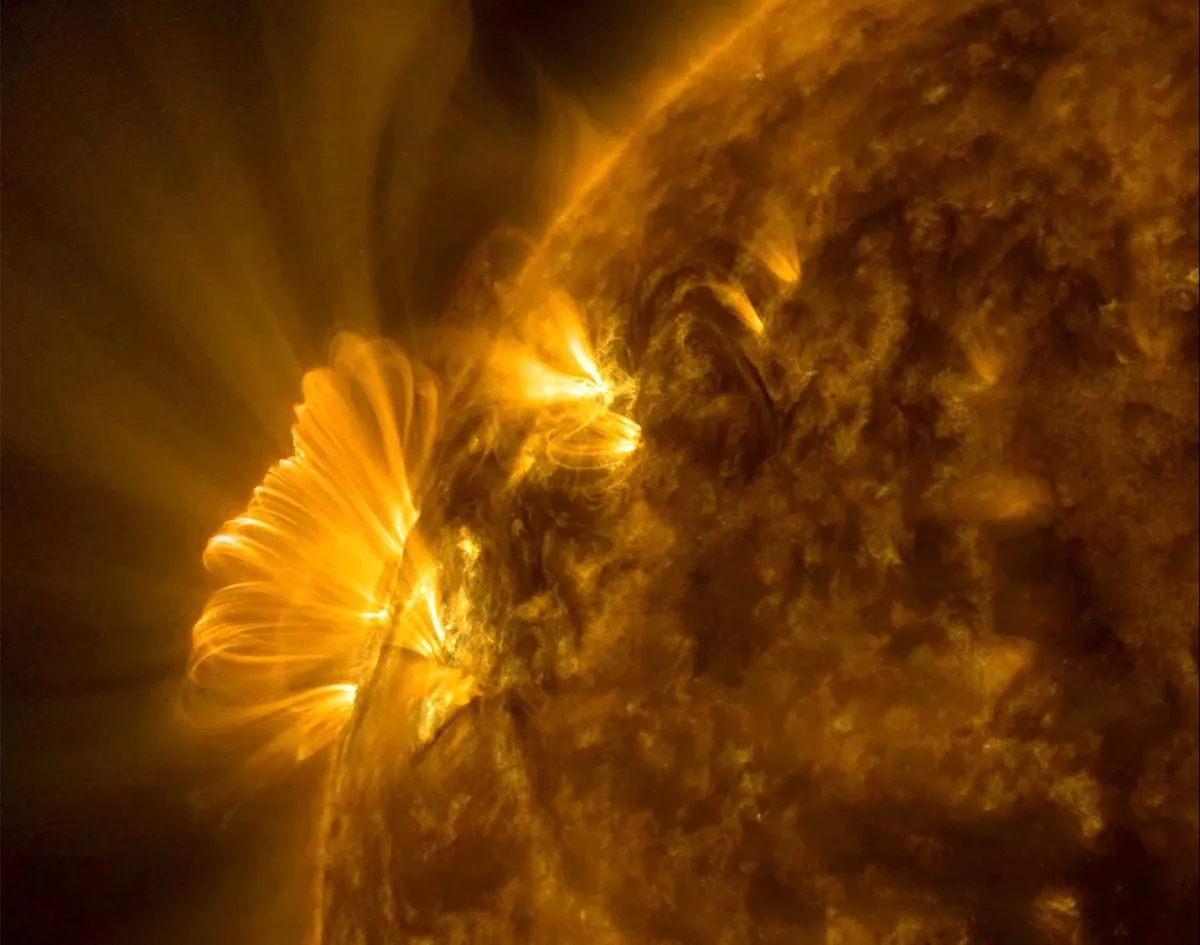
The Sun and stars are in a different state of matter than the solids, liquids and gases with which we are familiar.They consist of ionised particles that are in the plasma state.
Just as it takes energy to break chemical bonds to make a liquid from a solid (melting), and a gas from a liquid (evaporation), the addition of considerably more energy separates the electrons from their atomic nuclei, to form an electron-ion plasma.
Plasmas are the natural state of matter when the energy per particle – proportional to their temperature– is high enough to keep electrons separated from their parent atoms.
Most objects in astronomy bright enough to be seen are in the plasma state.
Along with charged particles, plasmas are flooded with light that is emitted and absorbed by the moving charges.
We can consider a plasma to consist of electrons, ions and photons, the latter being small quanta of light.
As we will see, the large energy densities required to make these astronomical plasmas arise naturally in astronomy from the inexorable effects of gravity, after matter condensed out of the raw energy of the Big Bang.
The defining property of plasma is that it is made up of free charges – electrons and ions.
Electrical charges were familiar to philosophers from 600 BCE. But its effects were first experimentally quantified far later, by Charles- Augustin de Coulomb (1736-1806).
He developed the concept of an “electric field”, in which each electrical charge endows the space around it with a force that acts on other charges. The lines of force begin and end at electrical charges.
The freedom of charged particles in a plasma to move between random collisions, means that the plasma is an excellent electrical conductor.
Just as the electrons easily move along household copper wires to form a current, an orderly relative motion of free electrons and ions in a plasma constitutes an electric current inside the plasma itself.
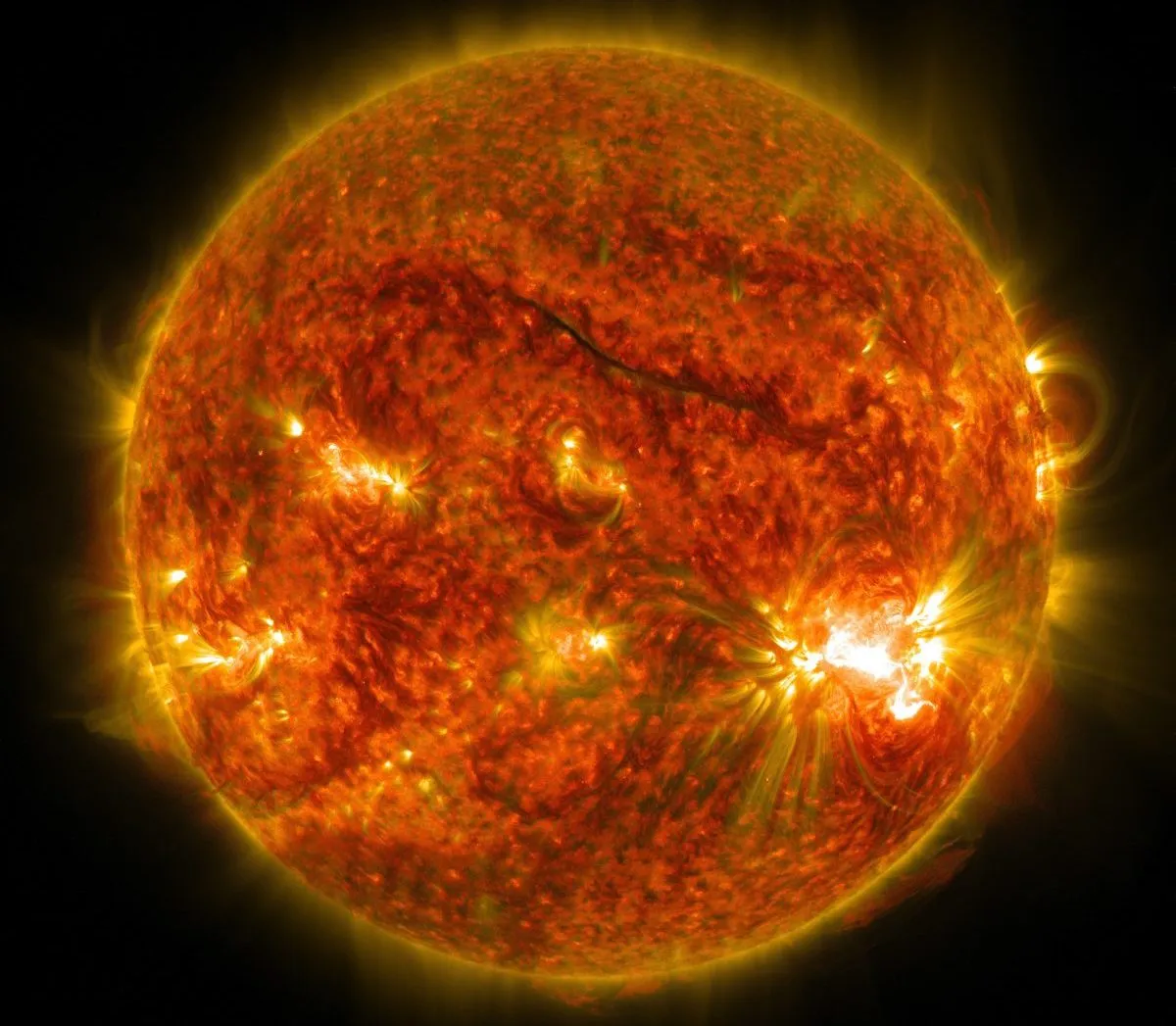
The Sun’s corona conducts electricity like copper, but plasma is more like a fluid than a wire. Mercury is familiar to us as a liquid on Earth’s surface, with a conductive effiency of about one fiftieth of copper, and indeed laboratory experiments with liquid metals have helped inform us about the workings of the Sun.
In contrast, seawater supports electrical currents carried by electrons removed from and attached to ions of sodium and chlorine in solution.
These heavier ions are far less mobile than electrons, and so seawater has about one ten-millionth of the conductive efficiency of copper.
Both magnetic and electric fields exist in the Earth’s atmosphere, allowing birds to navigate, and sparks to occur through lightning.
But in the Sun, the electrons are so mobile that any large-scale electric fields are almost immediately shorted out, just as in a copper wire.
Any magnetic fields survive intact against short-outs, because no-one has found evidence for any magnetic charges, called “magnetic monopoles”.
Based upon historic experiments by Coulomb, Biot, Savart, Ampére, and Michael Faraday (1791-1867), James Clerk Maxwell (1831-1879) “unified” electricity with magnetism.
Experiments show that magnetic fields are generated not by monopoles, but by electric currents, i.e. the relative motion of positive and negative charges.
In turn, magnetic fields exert forces only when charges and field are in relative motion.
In the absence of monopoles, magnetic field lines have no beginning, and no end. Only two kinds of magnetic field lines can satisfy this condition.
One is that the magnetic field lines make up complete loops, such as those threading through and around bar magnets.
The other kind is in an “ergodic” state where field lines go on forever throughout the universe. The former concept is most useful for discussing a finite object like the Sun.

The Sun is important also because it is not quite as benign as some might believe.
The solar corona emits a steady stream of ultraviolet (UV) and X- radiation, as well as plasma emerging as the solar wind.
The interactions between dense solar plasma beneath the visible surface, and the magnetic fields that generate the corona, can eject intense and unpredictable bursts of high-energy radiation, jets, plasma and magnetic fields into interplanetary space.
The Sun is a machine that converts a small but important fraction of its benign power into variable energetic radiation, magnetism and particles.
It is fair to say that today the biggest problems in solar physics concern the dynamical interactions between solar plasma and its magnetic fields.
In passing the Earth, solar outbursts disturb Earth’s protective magnetic field, causing problems for electrical infrastructure, though leading to beautiful aurorae.
Our increasing technology-dependence makes our way of life vulnerable to sustaining damage as a result of the poorly-understood workings of our otherwise friendly neighbour.
This is an extract fromThe Sun: A Very Short Introduction by solar scientist Philip Judge, published by Oxford University Press. Extract used with permission.