Theoretical physics can be a tough subject for most of us to navigate.But some in the field of experimental physics, like Professor John Ellis, help the rest of us understand a bit more about the workings of the Universe, and the missing pieces of the puzzle.
Prof Ellis is appearing at the HowTheLightGetsIn festival at Hay-on-Wye, Saturday 25 May 2019.
BBC Sky at Night Magazine sat down with him to learn something new about the Universe around us.
What exactly is theoretical physics, and what is its overall aim?
The subject of my talk at HowTheLightGetsIn is 'What are are we? Where do we come from? Where are we going?' I would take that as the basic aim of theoretical physics.
Its aim is to understand how the Universe works, what it's made of, how it got to be in the state it is today, and what's going to happen in the future.
In order to do that, we have to figure out the fundamental laws governing the behaviour of the Universe: what's possible and what's not possible.
The everyday business of theoretical physicists is to propose laws, propose ways of testing laws, interpret experiments, all in the context of what we understand and what we don't understand.
We are attempting to understand, if you like, the rule book for the Universe.
For me, the objective is not just to understand the rule book, but to figure out what happened in the early Universe, what it's made of, and what ultimately will happen.

Those of us interested in astronomy might think that questions about the origin and the state of the Universe are the concerns of astronomers and cosmologists. How do theoretical physicists approach these problems?
The behaviour of the Universe in the large is governed as far as we can tell by Einstein's equations of general relativity, which I would point out were discovered by a theoretical physicist, not by a cosmologist!
A cosmologist or an astrophysicist tracks what's happening in the Universe; what's been happening.
But they don't, at least to my mind, provide explanations.
According to Einstein's equations, you might hold up both of your hands and say the left is gravity, and over on the right is all the matter in the Universe, which drives the behaviour of the gravity.
Our business is the stuff on the right: the matter that generates the gravity, and which is moved around by it.
Astrophysicists and cosmologists tell us there are large blobs of matter in the Universe; galaxies, galaxy clusters etc.
But as far as they can tell, there are no large blobs of antimatter.
We theoretical physicists knew from combining quantum theory with the special theory of relativity that there had to be this stuff called 'antimatter'.
But then the question becomes 'why does the Universe have blobs of matter but not any significant blobs of antimatter?'
We think we have a potential answer to that, which is the fact that the microphysical laws governing matter and antimatter are not quite equal and opposite, as originally thought.
In the laboratory we explore the very small microscopic differences, and what we theoretical physicists try to do is connect those very small differences with this mega difference in the Universe as a whole.
Where does the Standard Model fit into this, and how does theoretical physics relate to it?
The Standard Model is a mathematical framework that describes all the visible matter in the Universe.
If you want to know how a star works, what holds Earth together at the most basic level of our understanding, you go to the Standard Model, which tells you what particles there are, the forces between them, and you can then work with those fundamental laws and figure out something like how the Sun works, or how Earth works.
I would say that there's no confirmed experimental evidence in any laboratory for something that's not contained within the Standard Model.
However, we know there must be stuff beyond the Standard Model.
For example, consider the origin of the matter in the Universe and why matter dominates over antimatter.
That we cannot explain within the Standard Model. It would have to be some additional effect that maybe we can discover with our experiments. It can't be explained by the Standard Model alone.
With the Standard Model we can make stars and planets, but not galaxies.
Galaxies consist of lots of stars and gas and dust, held together by gravity.
But they need more gravity than what's generated by those stars, gas and dust.
So there must be something else generating more gravity, and that's what was named in the 1930s as dark matter.
Ever since then it's been a puzzle as to what that dark matter is, so we theoretical physicists propose models, we ask our experimental friends to do experiments to try to test our models.
We think there must be something beyond the Standard Model, but for the moment we don't know exactly what that is.

Is it fair to say that theoretical physics raises more questions than it answers?
I like to think of it like this. You could imagine knowledge like an expanding ball, growing all the time.
The more knowledge we acquire, the further out it expands.
But also, as the volume of knowledge increases, the surface area of open questions also increases.
I think it's more or less inevitable that the more we understand, the more we know, the more open questions there are.
One of the things you're going to be debating at the festival is the concept of parallel universes. Is this theory really what it seems; that there are an infinite number of different universes just like our own?
As far as we can tell, looking at the equations that we have to describe the very early behaviour of the Universe, it looks pretty much inevitable from the piece of Universe that we conceive with our telescopes, that there must be additional stuff that is invisible: too far away to see.
We can determine the laws of physics that hold in our Universe, and we know that the laws of physics governing stars that shone 10 billion years ago are the same to an extremely high accuracy as the laws that guide the stars in our own Galaxy today.
But we cannot from fundamental theory know whether those laws also apply to bits of the Universe beyond what we can actually see. If you go sufficiently far out, it might be very different.
That's the context in which people talk about multiverse and parallel universes and so on.
I have to take a somewhat conservative point of view and say that I'm not sure whether it's really terribly interesting to speculate about those parallel universes, or a multiverse.
We can't observe them; we can't figure out the laws that govern their behaviour.
It seems to me rather like medieval philosophers arguing about how many angels can stand on the head of a pin.
If you cannot answer that question in a scientific way, then for me it's not a terribly interesting question to ask.
My attitude, of course, is provisional. If somebody came along and said they had a fantastic new idea as to how you could actually determine whether or not there are parallel universes and the fundamental laws of physics within those parallel universes, then I would prick up my ears and would be very interested.

It sounds rather like the analogy of a hundred monkeys typing on a hundred typewriters forever and eventually writing the complete works of Shakespeare. Is part of the theory to do with the idea that if the Universe is ultimately infinite, anything is possible?
That certainly is a point of view and is related to what people call the anthropic principle.
For us to exist, the fundamental particles and their interactions must be just-so.
If you adjusted the relative strengths of the interactions or the masses of particles, then the behaviour of matter would be completely different.
And if you change it by a large amount, there's no matter at all and we don't exist.
One interpretation of that could be, as you said, hundreds or probably quintillions of monkeys bashing away on their laptops, producing laws of physics with different fundamental constants in them, and out of all those quintillions of monkeys, one of them just happened to bash in the numbers that lead to you and me having this conversation.
I find that somewhat unsatisfactory. I think that we should not just ascribe the origins of the numbers in the Standard Model to a bunch of monkeys, much as I love monkeys!
Why is there anything at all, rather than nothing?The honest answer to that is that we don't know.
I think we should try to look for some underlying interpretation of where those numbers come from.
I mentioned the origin of the matter in the Universe. There's this idea that we could relate the amount of matter in the Universe to some fundamental asymmetry between the behaviour of matter and antimatter, which we could hope to measure in the laboratory.
That was an idea that no-one had come up with before 1967. Before then, I think you would have had to say that amongst these quintillions of monkeys there was one monkey who bashed in the right fraction of matter and antimatter, and that's the Universe that we live in.
But now we have at least the potential to establish a more scientific explanation by relating this gross cosmological asymmetry between matter and antimatter to some sort of microscopic asymmetry that we can measure in the lab.
That would eventually provide us with some explanation of how that particular monkey hit that particular key and got that particular number.
You will also be debating the so-called 'God particle' at HowTheLightGetsIn. What is that and what does it mean?
We've said how there is a Standard Model that describes all the visible matter in the Universe.
But before 2012, there was one piece missing.
You could compare that to an arch with a capstone missing at the top. Without that capstone, the arch doesn't hold up, it just collapses.
It's the same with the Standard Model. There was a missing link, although I don't like that terminology.
This particle was something that was postulated on very theoretical grounds in 1964 by Peter Higgs.
Some other theorists had similar ideas although they didn't actually point out specifically that the arch needed this particular detectable particle capstone.
Initially these ideas were thought of as being very speculative, and you wouldn't get the time of day from the distinguished, grey-haired old professors if you started talking about the Higgs boson.
But gradually it came into the mainstream and in some sense it became the 'Holy Grail' of particle physics, to look for this missing capstone.
Sometime around 1990 or so, a very distinguished experimental physicist called Leon Lederman wrote a book.
It's a very good book, with lots of anecdotes from his time as an experimental physicist: it's thoroughly worth reading.
He talked about this Higgs boson as being that 'goddamn particle', and his publisher said "you can't write about a 'goddamn particle'!"
So they called it the 'God particle', which I think is absolutely terrible. It has nothing whatsoever to do with God. It's just a particle, like other particles.
Okay, it occupies a particular piece of the arch, and if you removed the capstone the arch would collapse, but it's also true that if you removed one of the other stones in the arch it would collapse.
It's important, but I don't think one should over-stress it, except in a historical sense in that it was the last particle in the Standard Model to be discovered.
That's what gave it the importance. It's just a particle.

What are the implications of its discovery?
It completed our description of the visible matter in the Universe.
However, it left us lots of other questions. In particular there are a lot of questions related to the Higgs boson itself.
Is it really an elementary particle or is it a composite particle? Why does the Higgs boson have the mass that it does?
This goes back to what we were talking about before. As soon as you answer one question, you get a whole load of other questions!
Much of what we are doing at the Large Hadron Collider at the moment is trying to address those other questions: to see whether the Higgs boson behaves the way the Standard Model predicted, or whether there's some deviation.
Maybe there's a deviation associated with the fact that it's not truly an elementary particle.
Maybe there are other Higgs bosons. Maybe there's other physics that helps us understand not just where the particle masses come from, but why they have the values they do.
Is this all ultimately pointing back to the question "why does anything exist?"
Well, the other question is what do you mean by 'anything'?
If you mean "why is there matter in the Universe?" that's a question that is very much on our agenda.
Why is there any 'thing' rather than any anti-thing, or equal amounts of things and anti-things?
But you could be asking the question at a deeper level. Why is there anything at all, rather than nothing?
The honest answer to that is that we don't know.
What we do know is that all the Universe that we can see contains stuff that's expanding, and people often talk about an initial explosion.
It's not really an explosion, because explosions happen in one place. It's more of an event that occurred everywhere, simultaneously, as far as we can tell.
We can devise experiments that go back to very soon after this event happened, and we can devise theories about what happened, which we can then test in the laboratory, but those theories break down when we actually go to the event itself.
That means that theorists can have a field day speculating about whether there really was a time equal to zero.
If there was, what happened there, and what happened during the time before zero? Again, this is speculation that I find a little bit arid.
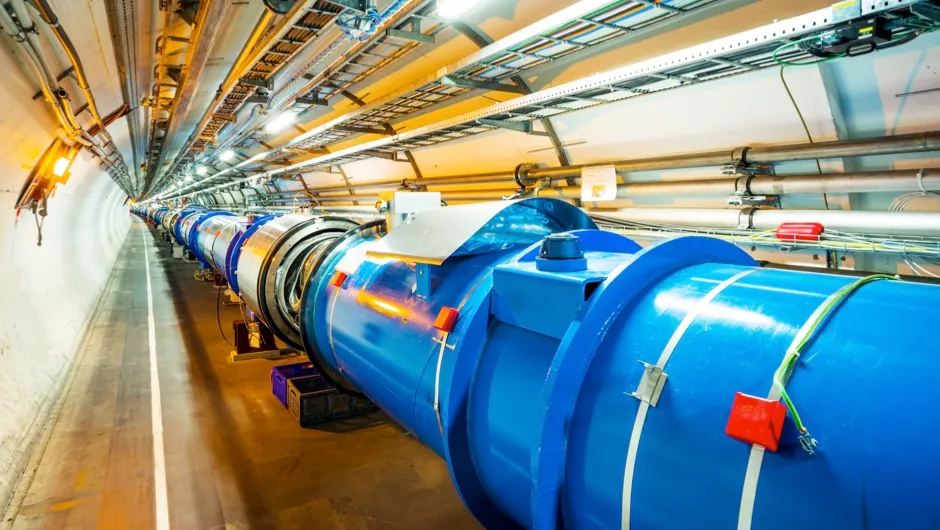
If we go back to the Big Bang, is there a chance that the laws of physics could have been such that no matter formed, that nothing formed and that there wouldn't ever have been anything at all in the Universe?
Again, it depends what one means by 'anything'.
We know that most of the individual particles in the Universe are not particles of matter.
They're not electrons and positrons and quarks and nuclei and so on and so forth.
They're actually photons - particles of light - and neutrinos.
You could imagine a Universe in which those were the only things. And if they were the only things then there wouldn't be any galaxies, there wouldn't be any planets.
So the question as to why there is some matter in addition to that multitude of photons and neutrinos: that gets us back to this question of the small asymmetry between matter and antimatter.
What is your reaction to the recently-published image of the supermassive black hole at the centre of galaxy M87? Does it have any influence on your own personal work?
First of all, it's a fantastic technological achievement, building a radio antenna that's basically as big as Earth!
It makes the Large Hadron Collider look a little bit little.
As a theoretical physicist I allow my ideas to float wherever, and so I mainly think about particles and mass - the stuff on the right, as we said.
I occasionally also allow myself to think about the stuff on the left.
One of the things that I'm thinking about right now is "what do these observations tell us about gravity?"
What makes a black hole 'black', is that it has a so-called event horizon, and when things fall in they get lost: gone forever, according to general relativity.
Hawking told us that's not quite true, because quantum effects can mean that some particles boil off in the form of quantum radiation, although we've never seen that.
But our people find that a bit unsatisfactory and they say maybe the story's a bit more complicated than that.
In particular, because quantum mechanics doesn't like to lose information, maybe there's some way in which what's going on inside the event horizon could communicate with what's happening outside.
That might modify this picture that we have of this football-like event horizon.
Maybe it's a little bit rough, or maybe it expands and contracts. Maybe it's a little bit deformed, as if Ronaldo had kicked it or something.
This is something that we can in principle test using these data.
The first round of data are admittedly rather crude, and initial probes of this deviation from the traditional general relativity/event horizon picture would not be possible with the current set of data.
But nevertheless it's something that we are thinking about.
John Ellis will be discussing his work in theoretical physics at HowTheLightGetsIn, Hay-on-Wye, Saturday 25 May 2019.