When the James Webb Space Telescope (JWST) launched on Christmas Day 2021, we knew its groundbreaking capabilities had the potential to rewrite the astronomy textbooks.
And this incredible spacecraft has not disappointed.
See the James Webb Space Telescope's latest images

The deployment of its giant segmented mirror and sun shield went without a hitch, its science instruments are operational and exceeding expectations, and the launch trajectory was so precise that there’s fuel to maintain its orbit for many years to come.
Right from its first observations, the James Webb Space Telescope has given astronomers new puzzles to solve and new questions to pursue.
In particular, it is challenging what we thought we knew about the early evolution of galaxies.

Impossible galaxies
JWST’s intriguing deep-field observations of faint light from the early, distant Universe reveal stars and galaxies that seem to be much larger than expected.
The CEERS (Cosmic Evolution Early Release Science) survey, led by Prof Steven Finkelstein of the University of Texas at Austin, used JWST’s NIRCam instrument to look back as far as the epoch of reionisation, just after the so-called dark ages of cosmic evolution, to study the structure of galaxies in the very early Universe.
It found more of them than predicted, and they appear bigger and brighter than expected.
According to our best models of how the infant Universe developed, they aren’t supposed to be there so early or look as they do.

Some of the first survey results have even indicated there are mature-looking disc galaxies reminiscent of our own Milky Way present as early as 10 billion years ago.
We were expecting a more chaotic picture, with predominantly irregular galactic structures interacting violently.
JWST’s advanced resolution, coupled with its ability to observe very distant early objects in infrared, has made us think again about how soon galaxies began to form and mature.
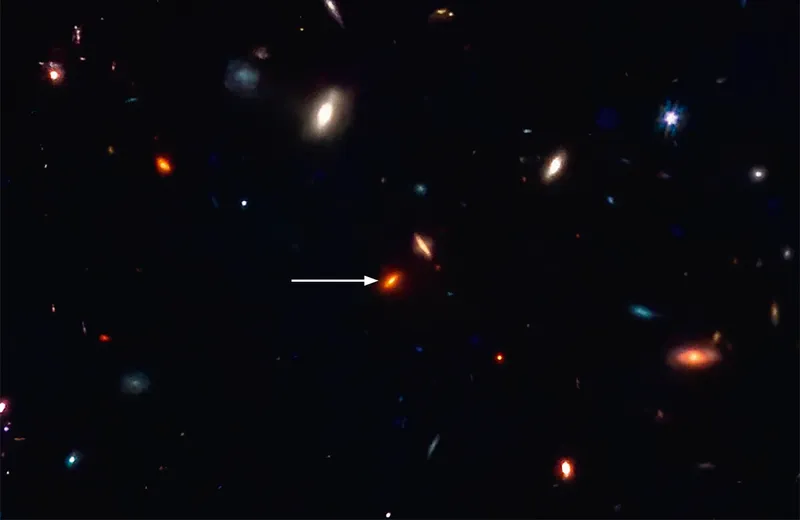
Early Universe is more mature than it should be
These findings challenge our current understanding of how the early Universe developed and pose some fascinating questions.
Were the properties of stars and galaxies in the infant Universe dramatically different to now?
Might the rate of star formation have been faster and more efficient in the early Universe?
Could the light from these early objects have been emitted in a way we’ve never seen before, because the chemical composition of the early Universe was different?
What does this mean for our best theoretical models of galaxy evolution?
Disc galaxies like our own may be where life could develop in the Universe, and we’re finding them much earlier than we thought.
Follow-up observations are ongoing, with larger datasets, complementary observations from other telescopes and spectroscopic analysis from JWST’s NIRSpec instrument to refine these early results and seek answers to the questions they have provoked.
"Using the Hubble Space Telescope we thought that disc galaxies were almost non-existent until the Universe was about six billion years old," says Christopher Conselice, professor of extragalactic astronomy at the University of Manchester, UK and a CEERS co-investigator.
"But these Webb results push the time these Milky Way-like galaxies form to almost the beginning of the Universe."

The discovery of unexpected galaxies doesn’t end here. Dr Tim Carleton and a team at Arizona State University have been working on another JWST survey, PEARLS (Prime Extragalactic Areas for Reionization and Lensing Science).
As part of this survey, JWST’s NIRCam has picked out an anomalous dwarf galaxy 98 million lightyears away, in an area where the team wasn’t expecting to see anything.
Even more interestingly, its behaviour doesn’t seem to fit with our current theories of galaxy evolution.
Dwarf galaxies are common enough, but this one isn’t doing what we might expect.
We would expect to see a galaxy like this interacting gravitationally with a bigger companion galaxy nearby, or actively forming new stars, or both.
This one, dubbed PEARLSDG, is doing neither.
It’s all alone and its stars are old, challenging our understanding of how galaxies work.
It raises the prospect that there could be many more of these anomalous galaxies out there, not doing what we expected, and we haven’t been able to detect them until now.

Expansion of the Universe
The James Webb Space Telescope has also been adding to what we know about the rate of the expansion of the Universe.
Back in 1929, the American astronomer Edwin Hubble presented the first observational evidence that the Universe is expanding in all directions.
Hubble proposed the ‘Hubble constant’ to express the rate of this expansion.
Astronomers have known since the 1990s that the rate at which the Universe is expanding is increasing, but can't explain why.
Explanations to account for this include the mysterious forces known as dark energy or dark matter, but perhaps it's some sort of unknown particle we don’t know about yet.
Numerous investigations have been undertaken to work out the nature of these unseen components of the Universe.
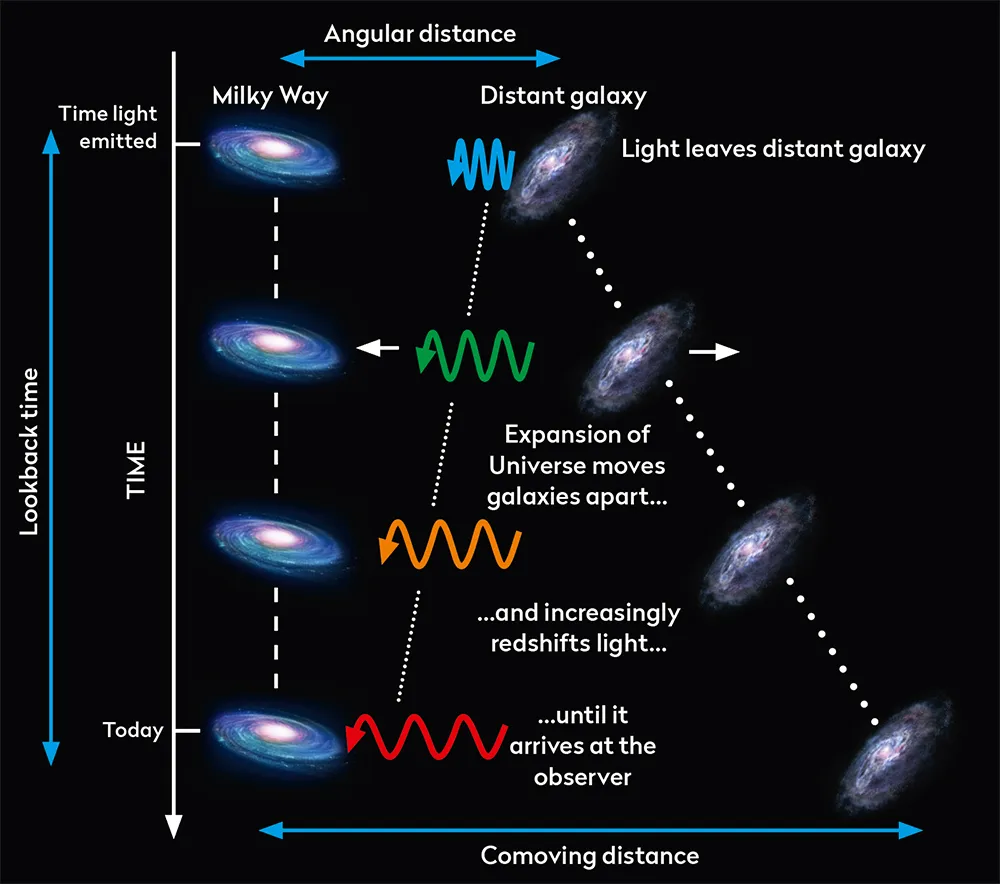
Cosmic acceleration can be measured by the distance of objects in the nearby Universe and how fast they're moving away from us.
Or by measuring fluctuations in the cosmic microwave background (heat left over from the Big Bang) linked to the density of matter in the early Universe.
You'd expect that these two methods would agree with one another, but instead measurements of the distant, early Universe suggest a slower rate of expansion than measurements of the nearby, more recent Universe.
The rate of expansion seems to vary depending on where you measure it.
This is called the Hubble tension and some have gone further and dubbed it the Hubble crisis.
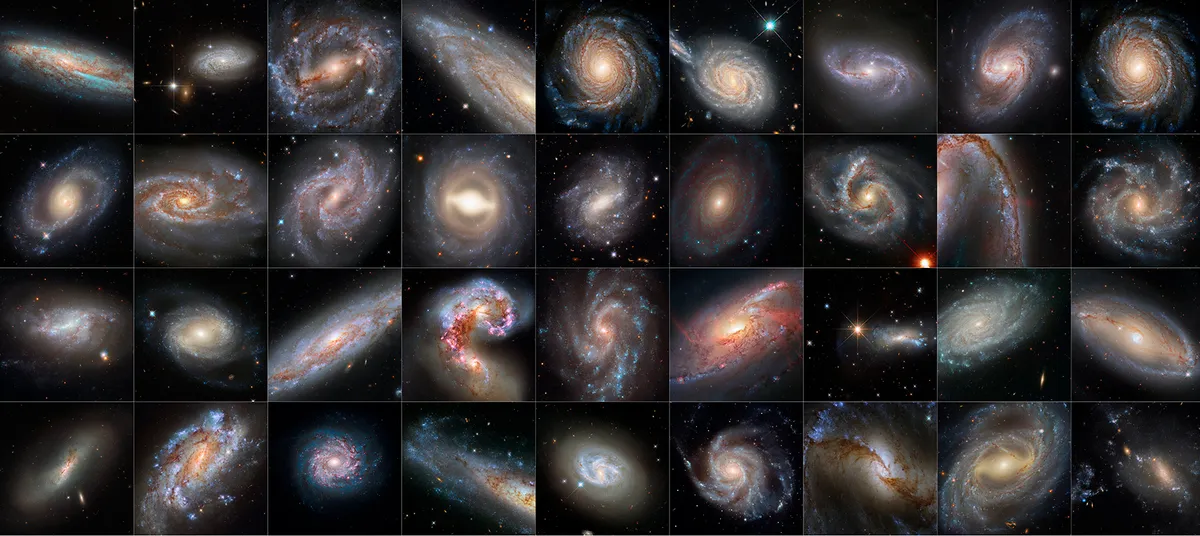
We need the Hubble constant to confirm the age of the Universe definitively and even predict what might happen in the future.
This is a big deal for cosmologists.
To put a number on the current expansion rate, we traditionally use measurements of Cepheid variables.
These are massive pulsating stars 100,000 times brighter than our Sun that act as useful distance markers in space.
Hubble has been making observations like this in our nearby Universe for decades, but there had been speculation that undetected measurement errors might be growing more pronounced as we looked deeper into the Universe.
Was crowding by other stars and dense dust clouds in the early Universe impacting the accuracy of more distant Cepheid luminosity and distance measurements?
Maybe this, or something like it, could explain the apparent discrepancy of the Hubble tension.

Enter the James Webb Space Telescope and the SH0ES (Supernovae and H0 for the Equation of State of Dark Energy) team led by Adam Riess at Johns Hopkins University.
Prof Reiss co-won the Nobel Prize for Physics in 2011 for the discovery of cosmic acceleration using data from the Hubble Space Telescope, and he and his team have used JWST to cross-check the Cepheid variable measurements across the whole distance range of Hubble’s observations.
With its enhanced precision to pick out Cepheids from other stars and its ability to see through the thick dust clouds obscuring them, JWST has confirmed definitively that the Hubble photometric observations of the Cepheids are correct.
We can now rule out a systematic measurement error.
So what else is causing the Hubble tension? There is the very real (and rather thrilling) possibility that we need some new physics to explain it.

How other missions can help
To assist with these new ideas, missions like ESA’s Euclid and NASA’s Nancy Grace Roman Telescope will conduct widefield surveys to tell us more about the influence of dark energy on expansion.
Roman is due to launch before the end of the decade, with a field of view 100x wider than Hubble.
One of its objectives will be to study how the distribution of galaxies and dark matter has changed throughout the history of the Universe.
Meanwhile, Euclid launched in July 2023 and is already exploring the composition and evolution of the ‘dark Universe’ on a cosmological scale.

All of this could help us resolve the Hubble tension and find an answer to this ongoing mystery.
"The measured mismatch between the Universe’s expansion rate now and 13 billion years ago, known as the Hubble tension, is an enormous mystery in modern cosmology," says Prof Adam Amara, head of maths and physics at the University of Surrey in the UK, who studies the evolution of the Universe and chairs the UK Space Agency Science Programme Advisory Committee.
"The recent exquisite observations from Webb confirming earlier findings from the Hubble Space Telescope deepen the mystery.
"Tantalisingly, they suggest that new exotic physics might be at play in the Universe – which we have yet to discover."
Why is the James Webb Space Telescope so good?

A more powerful telescope needs a bigger mirror.
At 6.5 metres, JWST’s mirror is the biggest ever flown and had to be made in segments, folded up inside the rocket for launch and unfurled and aligned in space.
This means JWST is 100x more sensitive than Hubble and can see fainter, more distant objects, further back in time.
There are bigger ground-based telescopes, but their observations are impeded by background interference on Earth.
To see the earliest objects, we need a very cold telescope, shielded from the Sun and Earth, pointing out into deep space.
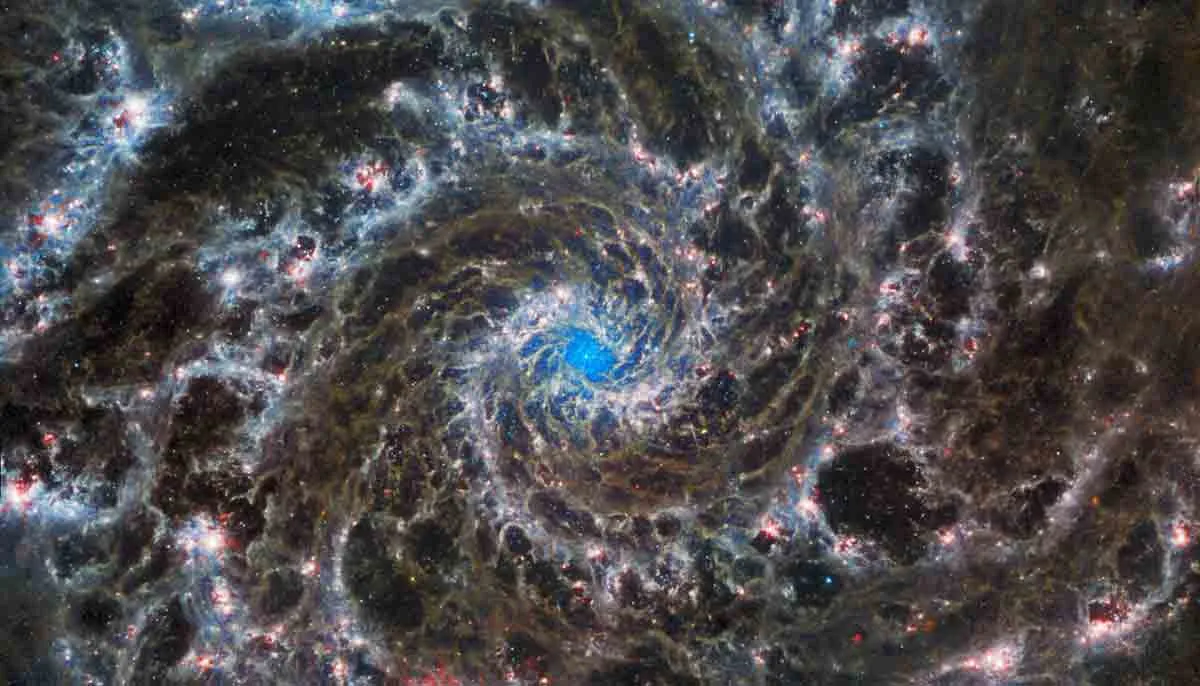
JWST is optimised to see in the near- and mid-infrared.
As light travels towards us from faraway objects, it gets stretched into longer wavelengths (red-shifted) due to the expansion of the Universe.
The light we see from a star near the beginning of the Universe took billions of years to reach us.
It may have started as ultraviolet or visible light, but by the time it reaches us it’s near- and mid-infrared light.
To see the early Universe, we need a telescope like JWST.
Its infrared capability also allows us to peer into dense dust clouds shrouding newly forming stars, giving us an inside view like never before.
This article appeared in the August 2024 issue of BBC Sky at Night Magazine