Light is one of those concepts we completely take for granted, but what actually is light?
It's not exactly an unknown concept: it crops up in every day conversation and we all know the apparent difference between light and darkness, but if asked to succinctly describe what light is, that's where most of us would struggle.
Here we'll take a look at light, what it is, how it operates and the different types.
But first, let's take a look at how scientists before us attempted to grasp this phenomenon; at once seemingly visible and yet intangible.
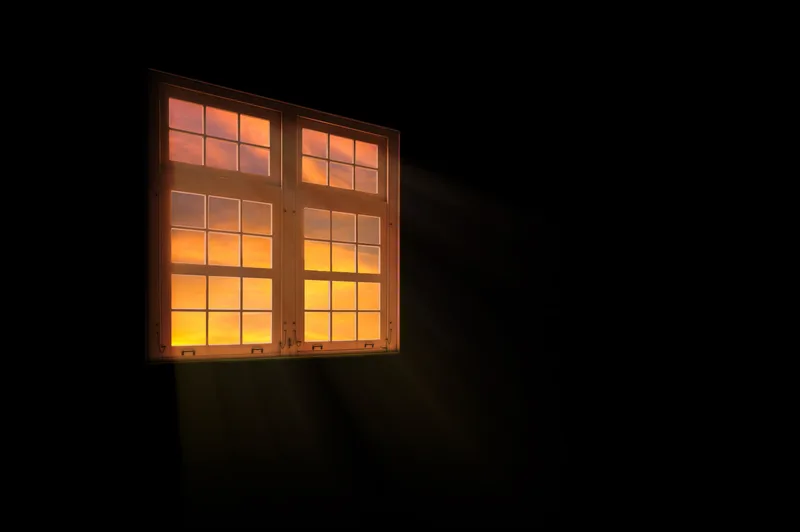
A history of working out what light is
The ancients argued long and hard about the nature of light.
Greek philosopher Empedocles somehow convinced the ancient world that the goddess Aphrodite lit a fire inside the human eye, making light shine out of it.
I know it is easy to scoff with the benefit of hindsight, but I suspect old Empedocles (who also believed that we breathe through our skin) was the loudest rather than the brightest philosopher in Ancient Greece.
More successful thinkers of antiquity worked out some of the principles of optics.
Euclid, Ptolemy and the wonderfully named Hero of Alexandria all had brilliant insights into the geometry of light rays, allowing them to design useful mirrors and predict where shadows would fall.
But, without the experiments and theories of modern physics, no one knew what light was made of until much more recently.
Even the great Isaac Newton got it wrong.
Because light travels in straight lines, he thought it must be made of particles called ‘corpuscles’, like tiny bullets that ricochet off mirrors and leave shadows in areas that are shielded from them.
Newton can be forgiven for believing this, because the idea works very nicely. It even seems to fit with the modern concept of photons, but actually, light’s not like that at all – we’ll talk more about photons later.
What is light? It's a wave!

Newton had a running argument with Dutch genius Christiaan Huygens, who believed that light was a kind of wave.
If you’ve ever visited a duck pond, you’ll know that waves can travel in straight lines too.
The bow-waves behind our feathered friends advance steadily across the surface until they reach the bank, where they can get reflected if the side of the pond is hard and flat, reminiscent of a mirror.
So waves sometimes travel in straight lines. But sometimes they don’t.

When the waves from a storm at sea arrive at a harbour entrance, they don’t just continue in a straight line to the quayside.
Instead, the lapping at the harbour-mouth creates ripples that spread out, disturbing all of the water
in the harbour.
This spreading out when waves pass through an opening is called ‘diffraction’.
It explains why you can hear people talking in the next room – because sound waves are diffracted when they pass through the doorway, so your ear is disturbed even when it doesn’t have a straight ‘line of sight’ to the speaker’s mouth.
Contradicting the corpuscle theory of light

Everyday experience seems to show that light, like particles, never gets diffracted.
You can’t see round corners and sunlight through a window only shines on the patch of carpet straight ahead, leaving the rest of the room in shadow.
So, for a hundred years, Newton’s corpuscle theory was accepted as fact. Which is a shame, because Huygens was right.
During Newton and Huygens’s lifetimes, the enormous speed of light was measured for the first time, by the astronomers Ole Rømer and Giovanni Cassini.
They noticed that light from the regular transits of Jupiter’s moon Io reaches Earth more quickly when Jupiter is close to us.

But this measurement didn’t shed any light (sorry) on the actual nature of the stuff.
The debate was finally settled at the turn of the 19th century by the brilliant Thomas Young
He demonstrated with his double-slit experiment that light doesn’t always travel in straight lines but, like water waves and sound waves, can spread out and make ‘diffraction patterns’.
These are bright patches of light in places that don’t have a straight line of sight to the light source.

Diffraction
It turns out that a wave doesn’t spread out very much when it passes through a wide aperture (a wide harbour mouth for water waves, or a wide doorway for sound waves), whereas narrow openings spread the waves out across a much wider angle.
In fact, in order to get a really noticeable amount of diffraction, the aperture needs to be not too much bigger than the wavelength (the spacing between one wave-crest and the next).
That is why the diffraction of light had never been noticed before Thomas Young shone a light on some really tiny openings – only a few hundredths of a millimetre wide.
In that one elegant experiment, he demonstrated that Newton, and almost everyone else since the dawn of time, had misunderstood the nature of light.

By measuring the amount of diffraction, Young was able to work out light’s wavelength.
He discovered that the ripples making up visible light are only about a two-thousandth of a millimetre apart.
So, to notice its wave-like properties at all, you need either very tiny or incredibly accurate optical equipment.
To notice the spreading of light waves passing through a 6-inch hole, for instance, you would need to be able to measure extraordinarily small angles of around one arcsecond.
But of course, dear reader, you probably own such an incredibly accurate piece of optical equipment with (if you’re lucky) a 6-inch hole at the front.
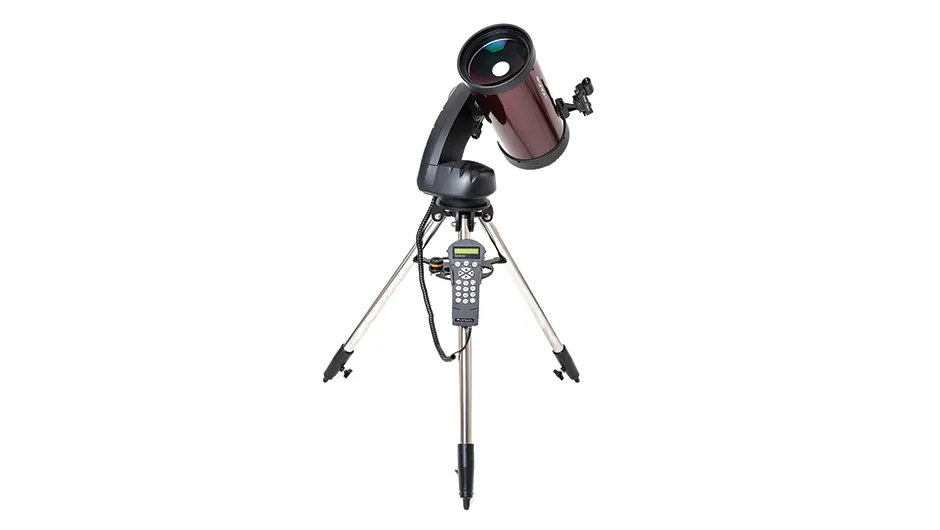
Light discoveries in the electric age
So light behaves just like the ripples on a pond. It’s a wave.
But a wave of what? Obviously not water.
And it's clearly not a wave of pressure in gas because, unlike sound, light can travel though airless space.
It was shy Victorian genius James Clerk Maxwell who finally found the answer while he was trying to understand the recently discovered curiosities called electricity and magnetism.
Michael Faraday had found that waving a magnet past a coil of copper wire generated electricity.
Meanwhile, André-Marie Ampère, a French aristo orphaned by the revolution, had shown that an electrical current flowing round a coil of wire generates magnetism.
Maxwell realised that the copper wire in those experiments was a distraction from what was really going on.
They revealed something profound about processes occurring in the empty space around the copper; processes that can happen even when no wire is present.
A vacuum can, in fact, be full of invisible magnetic and electric fields.
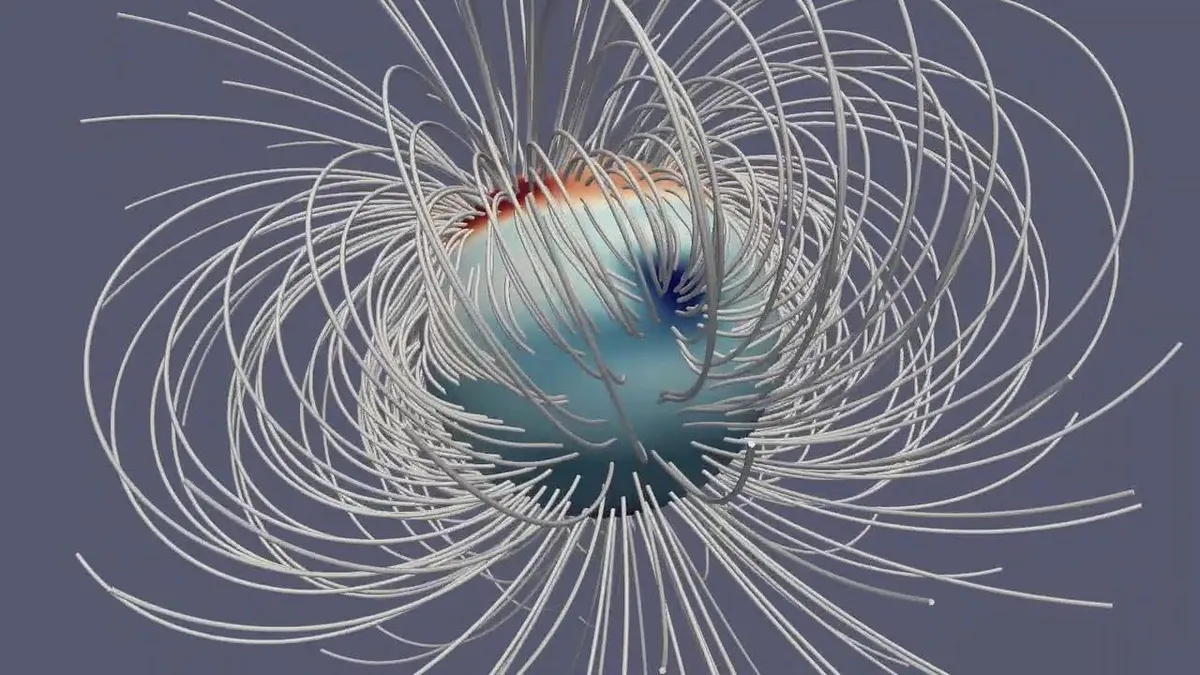
You can feel such a magnetic field when you try to force two repelling fridge magnets together, and an electric field when you rub a balloon and hold it near your hair.
Maxwell realised that any change in the magnetic field (for example, because you’re waving a magnet about) creates an electric field, and any change in the electric field creates a magnetic field.
So a disturbance in either field will ripple through space, with the electric and magnetic fields repeatedly generating each other, as they grow and collapse, on and on forever.
Using the data about the strengths of electric and magnetic forces published by Faraday and Ampère, Maxwell worked out how fast his proposed electromagnetic ripple should propagate and, being a physicist, he immediately recognised the answer as a very familiar number.
It was exactly the same as the speed of light.
What a ‘Eureka!’ moment that must have been, when Maxwell realised he had solved the age-old riddle of what light is made of.
So light is waves in electromagnetic fields. This theory explains the motion, colour and brightness of light (see ‘Types of light’ below).
Understanding the wave nature of light has allowed us to probe the structure of matter, even though its atoms and molecules are invisibly small, by interpreting the patterns of light that has been scattered by the materials.
James Clark Maxwell got it right. End of story... ish.

The discovery of photons
In the early 20th century, a pair of notorious troublemakers by the names of Max Planck and Albert Einstein spoiled Maxwell’s beautiful theory.
They argued that some extremely accurate measurements of very dim light proved that it is quantised.
In other words, that light comes in discrete amounts – little packets that are countable like particles.
They had opened a Pandora’s box, revealing the spooky, barely sane laws of quantum mechanics that underlie our superficially respectable physical world.
These countable quanta of light are called ‘photons’, but don’t mistake them for the corpuscles of Newton’s defunct theory.
The world’s most advanced cameras can detect individual photons.
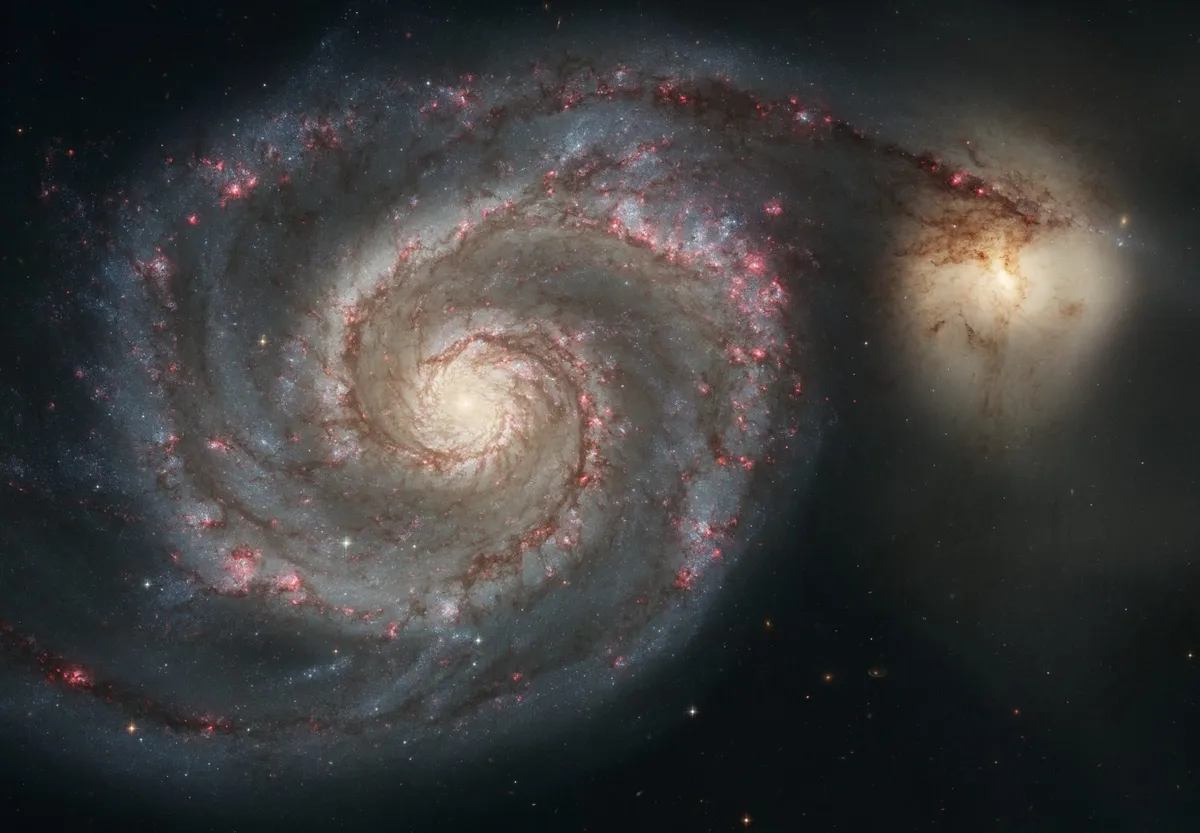
They build up an image from a set of dots that each arrive suddenly, giving the impression that photons are tiny hard particles crashing into the sensor.
But this impression is misleading. It may surprise you to learn what photons really are.
The discrete blips, which look like tiny particle impacts, are a result of the weird quantum-mechanical nature of the electromagnetic fields interacting with the measuring equipment.
Physicists still do not have a complete understanding of what goes on during this kind of measurement.
It needs a lot more research. But we do have a very accurate theory for the behaviour of those fields.
It’s based on Maxwell’s wave theory, which actually was not blown out of the water by Planck and Einstein’s discovery.
In fact, the quantum-mechanical laws of the Universe only require us to tweak one tiny aspect of Maxwell’s brilliant theory.
He was right that light is made of electromagnetic waves.
However, the laws of nature forbid a wave to have just any old amplitude. Instead, the amplitude is only allowed to have certain discrete values, separated by tiny increments.
There is a name for one of these increments in the amplitude of a light wave. It’s called a photon.
Types of light

James Clerk Maxwell showed that changes in electric and magnetic fields propagate through space as light waves.
An electric field surrounds any electrically charged object, such as a balloon that has been rubbed on clothes.
So, why don’t you see light shining out when you shake the balloon?
It’s only because you’re doing it too slowly.
You are actually creating very long radio waves, which are a colour of light that our eyes can’t see.
If you could shake an electric charge up and down about 500 million million times per second, you would create yellow light.
Our eyes are able to see electromagnetic waves in only a small range of wavelengths, from about three-quarters of a micron, which we see as red light, down to just over one-third of a micron, which is violet.
All other wavelengths of light are invisible to us and although they have names such as ‘X-rays’ and ‘microwaves’, they are just other colours in an infinite rainbow, shown below.
So that’s colour, but what about the other quality that characterises light: brightness?
Brightness is how we perceive the amplitude of the light wave – the amount of variation in electric and magnetic fields.
If you like the analogy with water waves, amplitude is the height of the ripples.
It’s related to the amount of energy in the wave.
The more effort you put into shaking the electric charges, the bigger the amplitude of the electromagnetic wave, and the brighter it will appear.
Scattered light

When light passes through a hole, the amount it diffracts depends on the size of the hole, relative to the wavelength.
So, observing the scattered light tells us the size of hole.
Light scattered from two apertures, as in the double-slit experiment, makes a more complex pattern, as the diffracted waves mix together.
The wider the spread of the pattern, the smaller are the dimensions of the scattering object.
In a crystal, the rows and columns of atoms create countless apertures.
Sending waves of a comparable wavelength through these gaps makes a diffraction pattern that can be measured, so we can work out the structure of the crystal.
Observing how light scatters can also reveal the structure of empty space.
If space is perfectly smooth, it won’t scatter light. If it is constructed from miniscule building-blocks, as many physicists suspect, it should scatter different wavelengths of light by different amounts – albeit tiny amounts, since the structure is much smaller than any observable wavelengths, blurring out the effects of the scattering.
Scientists using NASA’s Fermi Gamma-ray Space Telescope observed three photons with different wavelengths arriving at Earth simultaneously.
The photons emanated from a gamma-ray burst seven billion years ago – enough time to accumulate a noticeable difference in arrival times if one wavelength takes a slightly more wiggly path than another due to scattering.
The observed simultaneity puts a limit on the size of any fundamental units of space, if they exist at all.
This article appeared in the July 2013 issue of BBC Sky at Night Magazine