Gavin Hesketh is an experimental particle physicist currently working on the Large Hadron Collider.
His bookThe Particle Zoo seeks to answer some of the fundamental questions about our Universe.
BBC Sky at Night Magazine caught up with Gavin to find out more about the work he and his colleagues do, and why it is so important to our understanding of the cosmos.
Scroll down to below the interview to read an excerpt from the first chapter of The Particle Zoo.

What does a particle physicist do?
As a particle physicist, I do much the same as astronomers: try to learn more about the Universe we live in.
Astronomers use telescopes on mountain tops or even in space to look out to the cosmos, which is dominated by the force of gravity: massive objects like stars pulling at each other across immense distances.
Particle physicists on the other hand build our experiments deep underground to shield them from cosmic rays (subatomic particles from outer space bombarding Earth all the time), and look at the Universe on the smallest scales, a world dominated by the electromagnetic, weak and strong nuclear forces.
These are the forces that hold atoms together, and explain the structure of matter.
What is so exciting today is that we are connecting these different fields as we try to explain some of the mysteries of astronomy, like dark matter, using particle physics.
What can particle physics tell us about our own Solar System and Galaxy?
Over the last century, particle physics has definitely benefited from astronomy!
In the early 20th Century, cosmic rays were used to discover antimatter, as well as the first completely new particles: the muon and strange quark.
As the Sun fuses nuclei, it emits countless numbers of ghostly neutrino particles (billions pass through us every second and we don’t even notice) and studying these led to the 2015 Nobel Prize in physics being awarded to Takaaki Kahita and Arthur McDonald for discovering that they have tiny masses.
But particle physics has also given back.
Without it, we couldn’t explain how stars burn in the first place.
Particle physics is important to understand some of the most dramatic events in the Universe, like supernovae.
Neutrino experiments around the world now also double up as 'neutrino telescopes', looking for the highest energy particles and trying to understand what in the cosmos could be producing them.
So today there is more and more of an overlap between particle physics and astronomy.
What is being done at CERN and why it is relevant to our understanding of the Universe?
There are many experiments at CERN, but the biggest is definitely the Large Hadron Collider (LHC).
As the world’s most powerful particle accelerator, it accelerates particles.
Protons in fact, and it gets then very close to the speed of light before crashing them head on, and in these collisions we recreate some of the conditions of the very hot and energetic Universe just a fraction of a second after the Big Bang.
So the LHC can help to tell us how the Universe evolved from the Big Bang to the atoms, molecules, stars and planets that exist today.
And if, like all particle physicists hope, we turn up something unexpected, this will tell us something completely new about the Universe, and how it formed.
It might be a new force of nature, extra dimensions of space, or the elusive Dark Matter.
What is the smallest thing that we can currently observe?
Size is a funny idea in particle physics.
We are definitely looking at very small things, but when it comes to the fundamental particle, well, we have no idea how small they really are.
Even something everyday like the electron, the particle that powers all our TVs, phones, and so on.
Electrons are so small we haven’t been able to measure their size yet.
Perhaps if we are able to hit them hard enough, they will break apart into smaller things.
What is the Higgs boson? This video by Dave Barney of the CMS collaboration and Steven Goldfarb of the ATLAS collaboration reveals all. Animation by Jeanette Nørgaard for TED-Ed.
But there is no sign of this happening yet, and based on the experiments we have done so far, we know that all particles must be smaller than 10-18m,or less than a billionth of a billionth of a metre.
String theory suggests particles could be another billion billion times smaller; so small we may never be able to actually measure their size.
Below is an excerpt from Gavin’s new book, The Particle Zoo, published by Quercus.
Chapter 1: The Fundamental Nature of Reality
Beauty is perhaps a strange word to use when describing science, not least because it is so hard to define.
But we know it when we see it, and I was seventeen when I first saw the beauty in physics.
The way certain ideas draw an unexpected connection between two seemingly different topics.
How particular concepts appear over and over again.
And how all of this can be expressed in a few simple yet powerful equations.
When studying these ideas, it is hard not to feel that we can touch, even for just a moment, a deeper truth about the world around us.
The history of these ideas is a history of human creativity and imagination in discovering them – as well as of culture and politics, errors and luck, dead ends and breakthroughs.
But the beauty of science is that it can also transcend this: a single equation really can tell us something about the Universe that is as true today as it has been for billions of years, and can also tell us what will happen billions of years in the future.
But this story is not finished, and the future of science is about continuing to make sense of the world around us.
Particle physics is perhaps the most extreme form of this quest, trying to understand the universe by studying the smallest things in it: the fundamental particles.
The smallest pieces of matter.
The basic building blocks that make up you, me, and the whole world.
The same particles that form the Sun, our entire galaxy and the billions of other galaxies, and have existed for almost the entire history of the universe.
This book is about those particles.
It’s about what they are, how they behave, and the possibilities that exist in the world around us.
But it is also about where all these particles came from, how everything began, and how it might end.
These are some of the biggest questions we can ask, and the search for answers has revealed a universe that is far stranger than we could have imagined.
This is the story of what we know, of what we don’t, and of our quest to learn more.
It is the story of quarks and leptons, of bosons and symmetries, and of the biggest experiment in history studying the smallest things that we have discovered up to this moment.
Experiments really play a crucial role in this story.
Only by measuring the universe can we understand it, and the experiments I’ll describe vary from the biggest, the Large Hadron Collider, a huge 27-km particle accelerator studying the most extreme conditions ever created in a laboratory, to some of the less famous, like a giant tank of dry-cleaning fluid down a mine in South Dakota which studied the heart of the Sun.
Over a century of investigation has revealed an entire zoo of exotic particles, and led to the most successful scientific theory ever developed: the Standard Model.
This theory describes how particles behave, how they interact, and explains everything from how atoms form to how the Sun burns, and the way these particles shape the world around us.
The Standard Model has also predicted the result of every experimental test we have so far been able to devise – the real sign of scientific success.
As for me, well after getting hooked on physics I pursued a career in research at the energy frontier, the highest-energy particle accelerators in the world, finding new ways to test the Standard Model under more and more extreme conditions.
Now as a lecturer in Experimental Particle Physics at University College London, I was lucky enough to be working on the ATLAS experiment at the Large Hadron Collider in 2012 when we answered the final question about the Standard Model.
The missing piece that had been predicted almost forty years ago – and hunted ever since – was finally discovered: the Higgs boson.
This particle started out as a mathematical trick, but eventually became the key to the whole theory – it really holds the Standard Model together, and its discovery tells us about the deeper rules governing the behaviour of the universe.
We are really in a golden age for fundamental physics: we have the greatest experiment ever built pushing back the frontiers of human knowledge.
But what is most exciting is that we may be on the threshold of a completely new discovery, something much more significant than the Higgs boson, a discovery that could lead to a new revolution in our thinking about the universe and our place in it.
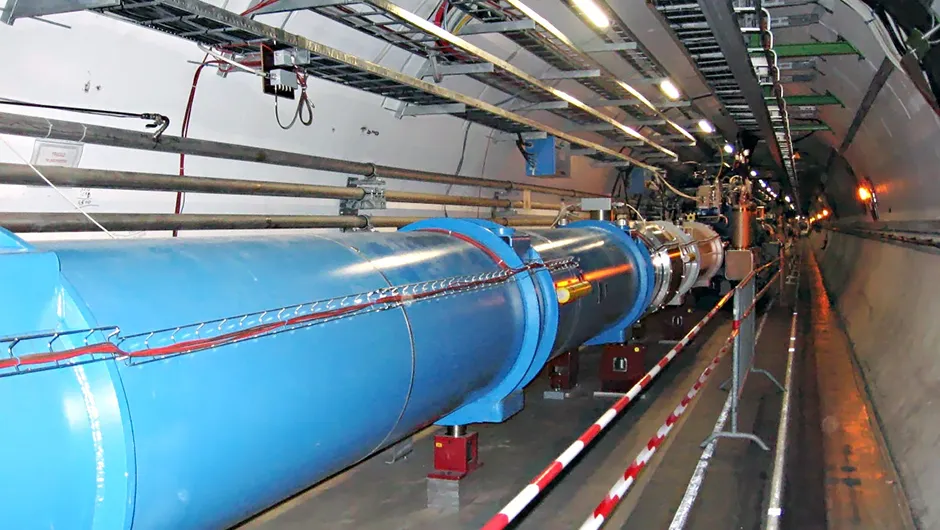
The atomic world
Before revolutionising physics, I need to introduce the main players in this show: the basic building blocks of the universe, and the forces that move them. This story begins with atoms.
We are taught at school that everything is made up of atoms, but this is still quite surprising when you stop to think about it.
All of the dazzling array of different stuff around us, solid objects like this book, liquids like water, all the creatures on Earth and the air we breathe, everything is made of atoms; we just don’t notice them because they are so small.
Take any everyday object, like a piece of paper, and start slicing it up.
You get smaller pieces of paper – a centimetre, a millimetre, a fraction of a millimetre in size.
If we had a magic knife that could keep on slicing, we would eventually cut the paper up into some molecules, and if we sliced those apart we would be left with atoms. It’s hard to really visualize how tiny an atom is, but a million of them would fit across the width of a single hair.
The idea of atoms, of basic building blocks of matter, existed in several ancient philosophical traditions, but the modern concept dates from the nineteenth century.
Solid experimental evidence proved that atoms were more than an idea, from Brownian motion (the jostling of tiny pollen grains by water molecules visible with a microscope) to the behaviour of gases and the way different chemicals combine to form new compounds.
In all of these experiments, atoms seem to behave like tiny solid marbles – something reflected in the word ‘atom’, which derives from the Greek for indivisible.
There are different types of atom, and they can stick together to form molecules – like two hydrogen and one oxygen atom making H2O, or water. Molecules can stick together to form different materials, like water molecules forming ice.
Atoms and molecules can also move around and bounce off each other, and we perceive this motion as pressure and temperature: more bouncing means higher pressure, and faster bouncing means higher temperature.
If atoms behave like tiny marbles, then they must be a little solid lump of something, and it’s sensible to ask what that something is – this is the search for the fundamental nature of reality after all.
However, because atoms are far too small to be seen directly, it required some new experiments in the early twentieth century to learn more about them.
We now know that atoms are not indivisible, but consist of a cloud of electrons orbiting a small, heavy nucleus made up of protons and neutrons – a little bit like the planets orbiting the Sun in our solar system.
Building on this analogy, our solar system has 8 planets, so we can compare it to an atom with 8 electrons: an oxygen atom.
If we could take one oxygen atom and enlarge it almost a million billion billion times, then the nucleus would be roughly the size of our Sun, and the rest of the atom would also look fairly similar to the solar system, just a bit bigger: the closest electrons would orbit a little way beyond Jupiter; the furthest electrons would extend far beyond Neptune.
Just as the solar system is a few small planets in millions of kilometres of empty space, an atom is just a few electrons orbiting the tiny central atomic nucleus.
This is one of the most surprising things about atoms: they are almost entirely empty, but can still stick together to form all of the solid objects around us.
This is thanks to the way electrons in neighbouring atoms interact, and the first clue that while particles are important, it is their interactions that really matter.
Now, just as we asked what an atom is made of, we can ask what the particles inside atoms are made of, and we now know that protons and neutrons are made up of smaller particles called quarks.
Two kinds of quarks, which we call the ‘up’ and the ‘down’ quarks, combine in different ways: up, up, down make a proton, and down, down, up make a neutron.
Everything is made of atoms, and atoms are made of just these three particles: electrons, up quarks and down quarks.
As far as we can tell, these quarks and electrons really are fundamental: they do not appear to be made of anything smaller.
They may be the basic building blocks of the universe.
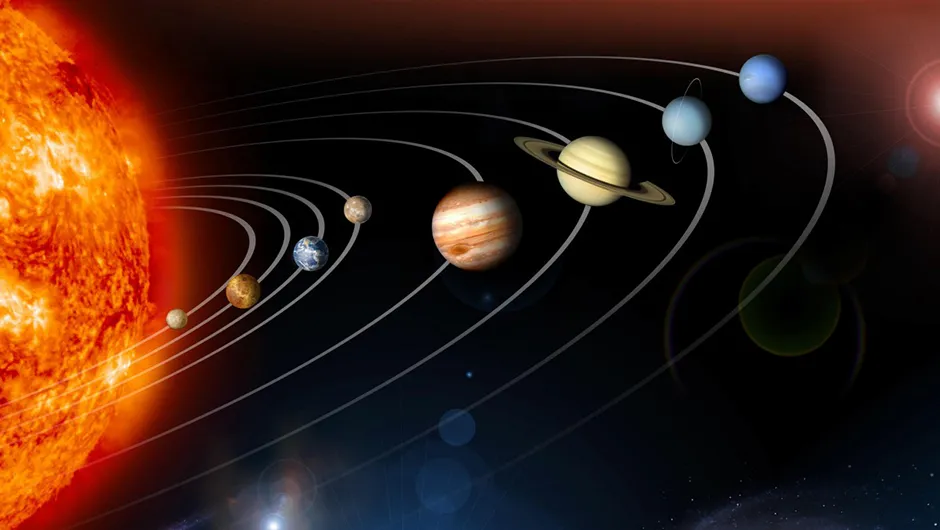
From atoms to particles
A fundamental particle is quite a strange thing.
Take an electron: it is not a small lump of some material, because we could always ask what that material is, and an electron would not be fundamental if it is made of something else.
The electron just . . . is.
We don’t know how big it really is, and in the mathematics of particle physics, all fundamental particles are treated as if they are infinitely small.
They are a collection of different properties like mass, electric charge and so on, but with no apparent physical size.
With such strange objects, it is probably no surprise that they behave in strange ways: they live in the unusual world of quantum mechanics.
This theory was developed in the 1920s, and was a true revolution in our understanding of the universe – it describes how these particles behave, and it is very different from the world as we know it.
Chapter 2 explores quantum mechanics and the randomness and uncertainty that make particles odd.
The true nature of fundamental particles is still something of a mystery. Sometimes they behave like solid little lumps, but at other times as a kind of fuzzy cloud, like a tiny fly constantly buzzing around.
Trapping an electron in an orbit around an atom is like trapping this fly in a bottle, and it will zip around all over the place, completely unlike the planets in the solar system, which travel in nice regular orbits.
But the failure of the solar-system model of the atom was just the first of many surprises about the fundamental particles, which can travel backwards in time, bend the laws of physics, pop in and out of existence and turn into entirely different particles.
While the fundamental particles all behave strangely, they do at least have some things in common, and we use these similarities to organise the subatomic world.
Electrons are a type of particle called a fermion, named after Enrico Fermi (who also has a space telescope, a particle physics laboratory, a chemical element, a few streets and several nuclear reactors named in his honour).
We know of 12 different fermions, which include the up and down quarks as well as the electron, and these are the ‘matter particles’, the particles that make up the solid things in the universe.
The basic characteristic of all fermions is that their fuzzy clouds don’t overlap, but stack up instead like little plastic building blocks – the stacking of electrons in atoms gives different chemical properties to different elements, and structure to the world around us.
These fundamentally strange particles make up everything around us, but at the same time are hard to pin down.
They are objects with no size, yet still take up space. We smash them together in particle accelerators to study how they behave, but the closer we look, the stranger they are.
And in the rest of this book I’ll explore some of that strangeness, and what it tells us about nature on the most basic level.